User:Nwbeeson/mySandboxx
The partisan mix of congressional delegations in the United States, 109th Congress, prior to the November 2006 elections, favors the Republican Party in both the United States House of Representatives and the United States Senate.
House of Representatives
[edit]
States | Republican Senators |
Democratic Senators |
Republican Representatives |
Democratic Representatives |
% Republican | % Democratic |
---|---|---|---|---|---|---|
Nebraska | 100% | 0% | 3/0 | 3 | ||
Idaho | 100% | 0% | 2/0 | 2 | ||
New Hampshire | 100% | 0% | 2/0 | 2 | ||
Alaska | 100% | 0% | 1/0 | 1 | ||
Delaware | 100% | 0% | 1/0 | 1 | ||
Montana | 100% | 0% | 1/0 | 1 | ||
Wyoming | 100% | 0% | 1/0 | 1 | ||
Kentucky | 83% | 17% | 5/1 | 4 | ||
Iowa | 80% | 20% | 4/1 | 3 | ||
Oklahoma | 80% | 20% | 4/1 | 3 | ||
Indiana | 78% | 22% | 7/2 | 5 | ||
Arizona | 75% | 25% | 6/2 | 4 | ||
Kansas | 75% | 25% | 3/1 | 2 | ||
Virginia | 73% | 27% | 8/3 | 5 | ||
Florida | 72% | 28% | 18/7 | 11 | ||
Alabama | 71% | 29% | 5/2 | 3 | ||
Louisiana | 71% | 29% | 5/2 | 3 | ||
Ohio | 67% | 33% | 12/6 | 6 | ||
South Carolina | 67% | 33% | 4/2 | 2 | ||
Nevada | 67% | 33% | 2/1 | 1 | ||
New Mexico | 67% | 33% | 2/1 | 1 | ||
Utah | 67% | 33% | 2/1 | 1 | ||
Texas | 65% | 35% | 20/11 (1 vacancy) |
9 | ||
Pennsylvania | 63% | 37% | 12/7 | 5 | ||
Michigan | 60% | 40% | 9/6 | 3 | ||
Connecticut | 60% | 40% | 3/2 | 1 | ||
Colorado | 57% | 43% | 4/3 | 1 | ||
Missouri | 56% | 44% | 5/4 | 1 | ||
Georgia | 54% | 46% | 7/6 | 1 | ||
North Carolina | 54% | 46% | 7/6 | 1 | ||
United States | 53% | 47% | 231/201 | 30 | ||
Minnesota | 50% | 50% | 4/4 | 0 | ||
Wisconsin | 50% | 50% | 4/4 | 0 | ||
Mississippi | 50% | 50% | 2/2 | 0 | ||
New Jersey | 50% | 50% | 6/6 (1 vacancy) |
0 | ||
Vermont | 0% | 0% | 0/0 (1 independent) |
0 | ||
Illinois | 47% | 53% | 9/10 | -1 | ||
Tennessee | 44% | 56% | 4/5 | -1 | ||
California | 38% | 62% | 20/33 | -13 | ||
West Virginia | 33% | 67% | 1/2 | -1 | ||
Washington | 33% | 67% | 3/6 | -3 | ||
New York | 31% | 69% | 9/20 | -11 | ||
Arkansas | 25% | 75% | 1/3 | -2 | ||
Maryland | 25% | 75% | 2/6 | -4 | ||
Oregon | 20% | 80% | 1/4 | -3 | ||
North Dakota | 0% | 100% | 0/1 | -1 | ||
South Dakota | 0% | 100% | 0/1 | -1 | ||
Hawaii | 0% | 100% | 0/2 | -2 | ||
Maine | 0% | 100% | 0/2 | -2 | ||
Rhode Island | 0% | 100% | 0/2 | -2 | ||
Massachusetts | 0% | 100% | 0/10 | -10 | ||
State ranked in partisan order | Percentage Republicans |
Percentage Democrats |
Republican/ Democrat |
Republican seat plurality |
Senate
[edit]
State ranked in partisan order | Percentage Republicans |
Percentage Democrats |
Republican/ Democrat |
Republican seat plurality |
---|---|---|---|---|
Alabama | 100% | 0% | 2/0 | 2 |
Alaska | 100% | 0% | 2/0 | 2 |
Arizona | 100% | 0% | 2/0 | 2 |
Georgia | 100% | 0% | 2/0 | 2 |
Idaho | 100% | 0% | 2/0 | 2 |
Kansas | 100% | 0% | 2/0 | 2 |
Kentucky | 100% | 0% | 2/0 | 2 |
Maine | 100% | 0% | 2/0 | 2 |
Mississippi | 100% | 0% | 2/0 | 2 |
Missouri | 100% | 0% | 2/0 | 2 |
New Hampshire | 100% | 0% | 2/0 | 2 |
North Carolina | 100% | 0% | 2/0 | 2 |
Ohio | 100% | 0% | 2/0 | 2 |
Oklahoma | 100% | 0% | 2/0 | 2 |
Pennsylvania | 100% | 0% | 2/0 | 2 |
South Carolina | 100% | 0% | 2/0 | 2 |
Tennessee | 100% | 0% | 2/0 | 2 |
Texas | 100% | 0% | 2/0 | 2 |
Utah | 100% | 0% | 2/0 | 2 |
Virginia | 100% | 0% | 2/0 | 2 |
Wyoming | 100% | 0% | 2/0 | 2 |
United States | 55% | 44% | 55/44 | 11 |
Colorado | 50% | 50% | 1/1 | 0 |
Florida | 50% | 50% | 1/1 | 0 |
Indiana | 50% | 50% | 1/1 | 0 |
Iowa | 50% | 50% | 1/1 | 0 |
Louisiana | 50% | 50% | 1/1 | 0 |
Minnesota | 50% | 50% | 1/1 | 0 |
Montana | 50% | 50% | 1/1 | 0 |
Nebraska | 50% | 50% | 1/1 | 0 |
Nevada | 50% | 50% | 1/1 | 0 |
New Mexico | 50% | 50% | 1/1 | 0 |
Oregon | 50% | 50% | 1/1 | 0 |
Rhode Island | 50% | 50% | 1/1 | 0 |
South Dakota | 50% | 50% | 1/1 | 0 |
Vermont | 0% | 50% | 0/1 (1 independent) |
-1 |
Arkansas | 0% | 100% | 0/2 | -2 |
California | 0% | 100% | 0/2 | -2 |
Connecticut | 0% | 100% | 0/2 | -2 |
Delaware | 0% | 100% | 0/2 | -2 |
Hawaii | 0% | 100% | 0/2 | -2 |
Illinois | 0% | 100% | 0/2 | -2 |
Maryland | 0% | 100% | 0/2 | -2 |
Massachusetts | 0% | 100% | 0/2 | -2 |
Michigan | 0% | 100% | 0/2 | -2 |
New Jersey | 0% | 100% | 0/2 | -2 |
New York | 0% | 100% | 0/2 | -2 |
North Dakota | 0% | 100% | 0/2 | -2 |
Washington | 0% | 100% | 0/2 | -2 |
West Virginia | 0% | 100% | 0/2 | -2 |
Wisconsin | 0% | 100% | 0/2 | -2 |
See also
[edit]Category:Legislative branch of the United States government Category:Members of the United States Congress Category:Politics of the United States Category:Political office-holders in the United States
black universities table
[edit]College name | Percent African American | Percent Other |
---|---|---|
Bluefield State College | 13Cite error: A <ref> tag is missing the closing </ref> (see the help page). |
75 |
West Virginia State University[2] | 17 | 72 |
Kentucky State University | 64 | 34 |
Delaware State University | 70 | 25 |
Lincoln University (Pennsylvania)[3] | 79 | 18 |
University of the District of Columbia[4] | 74 | 17 |
Elizabeth City State University | 81 | 17 |
Fayetteville State University | 78 | 16 |
Winston Salem State University[5] | 81 | 16 |
Xavier University of Louisiana[6] | 74 | 13 |
North Carolina A&T State University[7][8] | 88 | 12 |
Mammals Distinguishing features
[edit]ALl descendants of the common ancestor of Sinoconodon and extant monotremes, marsupials, and plancentals.(p. 1)
dentary condyle to the qwuamosal glenoiid in the jaw hinge (p. 1) presence of the petrosal promontiorium (p. 1)
Check out the goo
NASA Rocket
[edit]
A liquid-propellant rocket or a liquid rocket is a rocket engine that uses propellants in liquid form. Liquids are desirable because their reasonably high density allows the volume of the propellant tanks to be relatively low, and it is possible to use lightweight centrifugal turbopumps to pump the propellant from the tanks into the combustion chamber, which means that the propellants can be kept under low pressure. This permits the use of low-mass propellant tanks, resulting in a high mass ratio for the rocket.
An inert gas stored in a tank at a high pressure is sometimes used instead of pumps in simpler small engines to force the propellants into the combustion chamber. These engines may have a lower mass ratio, but are usually more reliable.[9] : 186, 187 and are therefore used widely in satellites for orbit maintenance.
Liquid rockets have been built as monopropellant rockets using a single type of propellant, bipropellant rockets using two types of propellant, or more exotic tripropellant rockets using three types of propellant. Bipropellant liquid rockets generally use a liquid fuel and a liquid oxidizer, such as liquid hydrogen or a hydrocarbon fuel such as RP-1, and liquid oxygen. The engine may be a cryogenic rocket engine, where the fuel and oxidizer, such as hydrogen and oxygen, are gases which have been liquefied at very low temperatures.
In this schematic A is the cross sectional area of the nozzle on its longitudinal axis, The subscript e refers to the exit of the nozzle, and the subscript 0 refers to atmospheric conditions.[9]
Liquid propellant rockets can be throttled (thrust varied) in realtime, and have control of mixture ratio (ratio at which oxidizer and fuel are mixed); they can also be shut down, and, with a suitable ignition system or self-igniting propellant, restarted.
Liquid propellants are also sometimes used in hybrid rockets, in which a liquid oxidizer is combined with a solid fuel.[9] : 354–356
History
[edit]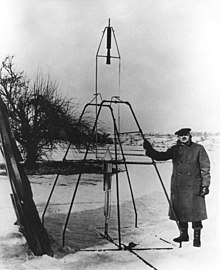
The idea of liquid rocket as understood in the modern context first appears in the book The Exploration of Cosmic Space by Means of Reaction Devices,[10] by the Russian schoolteacher Konstantin Tsiolkovsky. This seminal treatise on astronautics was published in 1903, but was not distributed outside of Russia until years later, and Russian scientists paid little attention to it.
During the 19th century, the only known developer of liquid propellant rocket engine experiments was Peruvian scientist Pedro Paulet, who is considered one of the "fathers of aeronautics.".[11] However, he did not publish his work. In 1927 he wrote a letter to a newspaper in Lima, claiming he had experimented with a liquid rocket engine while he was a student in Paris three decades earlier. Historians of early rocketry experiments, among them Max Valier and Willy Ley, have given differing amounts of credence to Paulet's report. Paulet described laboratory tests of, but did not claim to have launched a liquid rocket.
The first flight of a liquid-propellant rocket took place on March 16, 1926 at Auburn, Massachusetts, when American professor Dr. Robert H. Goddard launched a vehicle using liquid oxygen and gasoline as propellants.[12] The rocket, which was dubbed "Nell", rose just 41 feet during a 2.5-second flight that ended in a cabbage field, but it was an important demonstration that liquid-fueled rockets were possible. Goddard proposed liquid propellants about fifteen years earlier and began to seriously experiment with them in 1921.
After Goddard's success, German engineers and scientists became enthralled with liquid fuel rockets and designed and built rockets, testing them in the early 1930s in a field near Berlin.[13] This amateur rocket group, the VfR, included Wernher von Braun who became the head of the army research station that secretly built the V-2 rocket weapon for the Nazis. The German-Romanian Hermann Oberth published a book in 1922 suggesting the use of liquid propellants.
After World War II the American government and military finally seriously considered liquid-propellant rockets as weapons and began to fund work on them. The Soviet Union did likewise, and thus began the Space Race.
Principle of operation
[edit]All liquid rocket engines have tankage and pipes to store and transfer propellant, an injector system, a combustion chamber which is very typically cylindrical, and one (sometimes two or more) rocket nozzles. Liquid systems enable higher specific impulse than solids and hybrid rocket engines and can provide very high tankage efficiency.
Unlike gases, a typical liquid propellant has a density similar to water, approximately 0.7-1.4g/cm³ (except liquid hydrogen which has a much lower density), while requiring only relatively modest pressure to prevent vapourisation. This combination of density and low pressure permits very lightweight tankage; approximately 1% of the contents for dense propellants and around 10% for liquid hydrogen (due to its low density and the mass of the required insulation).
For injection into the combustion chamber the propellant pressure at the injectors needs to be greater than the chamber pressure; this can be achieved with a pump. Suitable pumps usually use centrifugal turbopumps due to their high power and light weight, although reciprocating pumps have been employed in the past. Turbopumps are usually extremely lightweight and can give excellent performance; with an on-Earth weight well under 1% of the thrust. Indeed, overall rocket engine thrust to weight ratios including a turbopump have been as high as 133:1 with the Soviet NK-33 rocket engine.
Alternatively, instead of pumps, a heavy tank of a high-pressure inert gas such as helium can be used, and the pump forgone; but the delta-v that the stage can achieve is often much lower due to the extra mass of the tankage, reducing performance; but for high altitude or vacuum use the tankage mass can be acceptable.
A liquid rocket engine (LRE) can be tested prior to use, whereas for a solid rocket motor a rigorous quality management must be applied during manufacturing to ensure high reliability.[14] A LRE can also usually be reused for several flights, as in the Space Shuttle.

Use of liquid propellants can be associated with a number of issues:
- Because the propellant is a very large proportion of the mass of the vehicle, the center of mass shifts significantly rearward as the propellant is used; one will typically lose control of the vehicle if its center mass gets too close to the center of drag.
- When operated within an atmosphere, pressurization of the typically very thin-walled propellant tanks must guarantee positive gauge pressure at all times to avoid catastrophic collapse of the tank.
- Liquid propellants are subject to slosh, which has frequently led to loss of control of the vehicle. This can be controlled with slosh baffles in the tanks as well as judicious control laws in the guidance system.
- They can suffer from pogo oscillation where the rocket suffers from uncommanded cycles of acceleration.
- Liquid propellants often need ullage motors in zero-gravity or during staging to avoid sucking gas into engines at start up. They are also subject to vortexing within the tank, particularly towards the end of the burn, which can also result in gas being sucked into the engine or pump.
- Liquid propellants can leak, especially hydrogen, possibly leading to the formation of an explosive mixture.
- Turbopumps to pump liquid propellants are complex to design, and can suffer serious failure modes, such as overspeeding if they run dry or shedding fragments at high speed if metal particles from the manufacturing process enter the pump.
- Cryogenic propellants, such as liquid oxygen, freeze atmospheric water vapour into very hard crystals. This can damage or block seals and valves and can cause leaks and other failures. Avoiding this problem often requires lengthy chilldown procedures which attempt to remove as much of the vapour from the system as possible. Ice can also form on the outside of the tank, and later fall and damage the vehicle. External foam insulation can cause issues as shown by the Space Shuttle Columbia disaster. Non-cryogenic propellants do not cause such problems.
- Non-storable liquid rockets require considerable preparation immediately before launch. This makes them less practical than solid rockets for most weapon systems.
Propellants
[edit]Thousands of combinations of fuels and oxidizers have been tried over the years. Some of the more common and practical ones are:
- liquid oxygen (LOX, O2) and liquid hydrogen (LH2, H2) – Space Shuttle main engines, Ariane 5 main stage and the Ariane 5 ECA second stage, the first and second stage of the Delta IV, the upper stages of the Ares I, Saturn V, Saturn IB, and Saturn I as well as Centaur rocket stage, the first stage and second stage of the H-II, H-IIA, H-IIB
- liquid oxygen (LOX) and kerosene or RP-1 – Saturn V, Zenit rocket, R-7 Semyorka family of Soviet boosters which includes Soyuz, Delta, Saturn I, and Saturn IB first stages, Titan I and Atlas rockets
- liquid oxygen (LOX) and alcohol (ethanol, C2H5OH) – early liquid fueled rockets, like German (World War II) A4, aka V-2, and Redstone
- liquid oxygen (LOX) and gasoline – Robert Goddard's first liquid-fuel rocket
- T-Stoff (80% hydrogen peroxide, H2O2 as the oxidizer) and C-Stoff (methanol, CH3OH, and hydrazine hydrate, N2H4•n(H2O as the fuel) – Walter Werke HWK 109-509 engine used on Messerschmitt Me 163B Komet a rocket fighterplane of (World War II)
- nitric acid (HNO3) and kerosene – Soviet Scud-A, aka SS-1
- inhibited red fuming nitric acid (IRFNA, HNO3 + N2O4) and unsymmetric dimethyl hydrazine (UDMH, (CH3)2N2H2) – Soviet Scud-C, aka SS-1-c,-d,-e
- nitric acid 73% with dinitrogen tetroxide 27% (=AK27) and kerosene/gasoline mixture (=TM-185) – various Russian (USSR) cold-war ballistic missiles (R-12, Scud-B,-D), Iran: Shahab-5, North Korea: Taepodong-2
- hydrogen peroxide and kerosene – UK (1970s) Black Arrow, USA Development (or study): BA-3200
- hydrazine (N2H4) and red fuming nitric acid – Nike Ajax Antiaircraft Rocket
- Aerozine 50 and dinitrogen tetroxide – Titans 2–4, Apollo lunar module, Apollo service module, interplanatary probes (Such as Voyager 1 and Voyager 2)
- unsymmetric dimethylhydrazine (UDMH) and dinitrogen tetroxide – Proton rocket and various Soviet rockets
- monomethylhydrazine (MMH, (CH3)HN2H2) and dinitrogen tetroxide – Space Shuttle orbiter's Orbital maneuvering system (OMS) engines and Reaction control system (RCS) thrusters.
One of the most efficient mixtures, oxygen and hydrogen, suffers from the extremely low temperatures required for storing hydrogen and oxygen as liquids (around 20 K or −253 °C)) and low fuel density (70 kg/m³), necessitating large and heavy tanks. The use of lightweight foam to insulate the cryogenic tanks led to tragedy for the Space Shuttle Columbia's STS-107 mission, as a piece broke loose, damaged its wing and caused it to break up and be destroyed on atmospheric reentry.
For storable ICBMs and interplanetary spacecraft, storing cryogenic propellants over extended periods is awkward and expensive. Because of this, mixtures of hydrazine and its derivatives in combination with nitrogen oxides are generally used for such rockets. Hydrazine has its own disadvantages, being a very caustic and volatile chemical as well as being toxic and carcinogenic. Consequently, hybrid rockets have recently been the vehicle of choice for low-budget private and academic developments in aerospace technology. Also the RP-1/LOX combination has become a popular choice for reliable and cost-sensitive commercial spaceflight applications.
Injectors
[edit]The injector implementation in liquid rockets determines the percentage of the theoretical performance of the nozzle that can be achieved. A poor injector performance causes unburnt propellant to leave the engine, giving extremely poor efficiency.
Additionally, injectors are also usually key in reducing thermal loads on the nozzle; by increasing the proportion of fuel around the edge of the chamber, this gives much lower temperatures on the walls of the nozzle.
Types of injectors
[edit]Injectors can be as simple as a number of small diameter holes arranged in carefully constructed patterns through which the fuel and oxidiser travel. The speed of the flow is determined by the square root of the pressure drop across the injectors, the shape of the hole and other details such as the density of the propellant.
The first injectors used on the V-2 created parallel jets of fuel and oxidizer which then combusted in the chamber. This gave quite poor efficiency.
Injectors today classically consist of a number of small holes which aim jets of fuel and oxidiser so that they collide at a point in space a short distance away from the injector plate. This helps to break the flow up into small droplets that burn more easily.
The main type of injectors are
- Shower Head type
- Self Impinging doublet type
- Cross impinging triplet type
- Centrifugal or Swirling type
- Pintle injector type
The Pintle injector permits good mixture control of fuel and oxidizer over a wide range of flow rates. The pintle injector was used on the Apollo Lunar Module engines See Descent Propulsion System and the current Merlin and Kestrel engines designed by SpaceX and used on Falcon 9 and planned Falcon Heavy rockets.
The Space Shuttle Main Engine uses a system of fluted posts, which use heated hydrogen from the preburner to vaporize the liquid oxygen flowing through the center of the posts[15] and this improves the rate and stability of the combustion process; previous engines such as the F-1 used for the Apollo program had significant issues with oscillations that led to destruction of the engines, but this was not a problem in the SSME due to this design detail.
Valentin Glushko invented the centrifugal injector in the early 1930s, and it has been almost universally used in Russian engines. Rotational motion is applied to the liquid (and sometimes the two propellants are mixed), then it is expelled through a small hole, where it forms a cone-shaped sheet that rapidly atomizes. Goddard's first liquid fuel engine used a single impinging injector. German scientists in WWII experimented with impinging injectors on flat plates, used successfully in the Wasserfall missile.
Combustion stability
[edit]To avoid instabilities such as chugging which is a relatively low speed oscillation the engine must be designed with enough pressure drop across the injectors to render the flow largely independent of the chamber pressure. This is normally achieved by using at least 20% of the chamber pressure across the injectors.
Nevertheless, particularly in larger engines, a high speed combustion oscillation is easily triggered, and these are not well understood. These high speed oscillations tend to disrupt the gas side boundary layer of the engine, and this can cause the cooling system to rapidly fail, destroying the engine. These kinds of oscillations are much more common on large engines, and plagued the development of the Saturn V, but were finally overcome.
Some combustion chambers, such as the SSME uses Helmholtz resonators as damping mechanisms to stop particular resonant frequencies from growing.
To prevent these issues the SSME injector design instead went to a lot of effort to vapourise the propellant prior to injection into the combustion chamber. Although many other features were used to ensure that instabilities could not occur, later research showed that these other features were unnecessary, and the gas phase combustion worked reliably.
Testing for stability often involves the use of small explosives. These are detonated within the chamber during operation, and causes an impulsive excitation. By examining the pressure trace of the chamber to determine how quickly the effects of the disturbance die away, it is possible to estimate the stability and redesign features of the chamber if required.
Engine cycles
[edit]For liquid propellant rockets four different ways of powering the injection of the propellant into the chamber are in common use.[16]
Generally speaking, pumping losses are small compared to the heat energy lost in the nozzle. For atmospheric use, high pressure engine cycles are desirable as it improves the efficiency of the nozzle. For vacuum use, pumps aren't usually required.
- pressure fed cycle – the propellants are forced in from pressurised (relatively heavy) tanks. The heavy tanks mean that a relatively low pressure is optimal. The pressurant used is frequently helium due to its lack of reactivity.
- expander cycle – cryogenic fuel is used to cool the walls of the combustion chamber and nozzle. Absorbed heat vaporizes and expands the fuel which is then used to drive the turbopumps before it enters the combustion chamber. No heat or propellant is lost, so efficiency is very high. Pump power and combustion pressure are constrained by available heat transfer.
- gas generator cycle – a small percentage of the propellants are burnt in a preburner to power a turbopump and then exhausted through a separate nozzle, or low down on the main one. This usually gives a small reduction in performance.
- staged combustion cycle – the high pressure outlet from the turbopump is fed back to power a burner which then powers the turbopump in a self-starting cycle. The still high pressure exhaust from the turbine is then fed directly into the main chamber, thus essentially all the energy goes through the nozzle, giving no pumping losses at all, and permitting very high pressures.
Engine cycle tradeoffs
[edit]Deciding on an engine cycle is one of the foremost steps to engine selection
Cycle type: | Gas generator (open cycle) | Expander cycle (closed cycle) | Staged-combustion (closed cycle) |
---|---|---|---|
Advantages | simple; low pressures; low inert mass; lower development cost | Good specific impulse; fair engine simplicity; no gas generator; possible small vehicle | best specific impulse; smaller thrust chamber size; possible smaller vehicle |
Disadvantages | slightly lower specific impulse | heavier engine; more expensive; increased complexity; heat transfer to the fuel limits available power to the turbine | more expensive; increased complexity |
Cooling
[edit]Injectors are commonly laid out so that a fuel-rich layer is created at the combustion chamber wall. This reduces the temperature there, and downstream to the throat and even into the nozzle and permits the combustion chamber to be run at higher pressure, which permits a higher expansion ratio nozzle to be used which gives a higher ISP and better system performance.[17] A liquid rocket engine often employs regenerative cooling, which uses the fuel or the oxidiser to cool the chamber and nozzle.
Ignition
[edit]Ignition can be performed in many ways, but perhaps more so with liquid propellants than other rockets a consistent and significant ignitions source is required; a delay of ignition (in some cases as small as) a few tens of milliseconds can cause overpressure of the chamber due to excess propellant. A hard start can even cause an engine to explode.
Generally, ignition systems try to apply flames across the injector surface, with a mass flow of approximately 1% of the full mass flow of the chamber.
Safety interlocks are sometimes used to ensure the presence of an ignition source before the main valves open; however reliability of the interlocks can in some cases be lower than the ignition system. Thus it depends on whether the system must fail safe, or whether overall mission success is more important. Interlocks are rarely used for upper, unmanned stages where failure of the interlock would cause loss of mission, but are present on the SSME, to shut the engines down prior to liftoff of the Space Shuttle. In addition, detection of successful ignition of the igniter is surprisingly difficult, some systems use thin wires that are cut by the flames, pressure sensors have also seen some use.
Methods of ignition include pyrotechnic, electrical (spark or hot wire), and chemical. Hypergolic propellants have the advantage of self igniting, reliably and with less chance of hard starts. In the 1940s, the Russians began to start engines with hypergolic fuel, then switch over to the primary propellants after ignition. This was also used on the American F-1 rocket engine on the Apollo program.
References
[edit]- ^ Apart No More? HBCUs Heading Into an Era of Change - HBCUConnect.com
- ^ "West Virginia State University". Retrieved 2010-12-01.
- ^ U.S. News & World Report Best Colleges, 2011 ed.Directory p252
- ^ U.S. News & World Report Best Colleges, 2011 ed.Directory p151
- ^ U.S. News & World Report Best Colleges, 2011 ed.Directory p234
- ^ U.S. News & World Report Best Colleges, 2011 ed.Directory p182
- ^ for 2013–14 school year
- ^ "Preeminence 2020: Embracing Our Past, Creating Our Future" (PDF). ncat.edu. North Carolina A&T. Retrieved 23 March 2014.
- ^ a b c Sutton, George P. (1963). Rocket Propulsion Elements, 3rd edition. New York: John Wiley & Sons. p. 25.
- ^ Russian title Issledovaniye mirovykh prostranstv reaktivnymi priborami (Исследование мировых пространств реактивными приборами)
- ^ "The alleged contributions of Pedro E. Paulet to liquid-propellant rocketry". NASA.
- ^ "Re-Creating History". NASA.
- ^ "The World's First Rocket Aerdrome", May 1931, Popular Mechanics
- ^ NASA:Liquid rocket engines, 1998, Purdue University
- ^ Sutton, George P. and Biblarz, Oscar, Rocket Propulsion Elements, 7th ed., John Wiley & Sons, Inc., New York, 2001.
- ^ "Sometimes, Smaller is Better".
- ^ Rocket Propulsion elements - Sutton Biblarz, section 8.1
External links
[edit]- An online book entitled ”How to Design, Build, and Test Small Liquid-Fuel Rocket Engines”
- The Heinkel He 176, worlds's first liquid-fuel rocket aircraft
Category:American inventions
Category:Rocket propulsion
Category:Rocket engines