User:Huginnscientia/Turtle brain evolution
I will add information about brain anatomy and neuroanatomy of turtles to the Turtle page on Wikipedia, under the Anatomy and Physiology section. There is no mention of the keyword’s “brain” or “neuron” on the page.
I will add information about the reptilian brain to the Brain page on Wikipedia, under the Reptiles subheading, under Anatomy heading. There is no information about reptilian brain evolution on that section.
![]() | This is the sandbox page where you will draft your initial Wikipedia contribution.
If you're starting a new article, you can develop it here until it's ready to go live. If you're working on improvements to an existing article, copy only one section at a time of the article to this sandbox to work on, and be sure to use an edit summary linking to the article you copied from. Do not copy over the entire article. You can find additional instructions here. Remember to save your work regularly using the "Publish page" button. (It just means 'save'; it will still be in the sandbox.) You can add bold formatting to your additions to differentiate them from existing content. |
To be added to Turtle page:
Anatomy and Physiology
[edit]Brain
[edit]General Anatomy
[edit]
The turtle brain is comprised of three distinct regions. First, the forebrain is comprised of olfactory sacs and bulbs, two hemispheres, cortex, dorsal ventricular ridge, optic chiasm, pineal body, and pituitary gland. Second, the midbrain is comprised of tectum, tegmentum, and isthmus. Third, the hindbrain contains optic lobes, cerebellum, choroid plexus, and medulla oblongata[1][2][3][4].
The turtle brain has twelve cranial nerves: I - olfactory, II - optic, III - oculomotor, IV - trochlear, V - trigeminal, VI - abducens, VII - facial, VIII - auditory, IX - glossopharyngeal, X - vagus, XI - spinal, and XII - hypoglossal nerves[3][2][4].
The cortex, located on the cerebral hemispheres, is subdivided in three cell layers and in three main areas - the medial, dorsal, and lateral cortices[4][5]. In turtles, the medial layer is comprised of a densely packed layer of neuronal cells[5], containing two principal classes of neurons: pyramidal cells, characterized by spiny apical dendrites and firing of long-duration action potentials; and stellate neurons, found in the subcellular and molecular layers, characterized by the lack of spiny dendrites and firing of short action potentials[6].
Function and Cognition
[edit]Cognition involves the ability to acquire information from the environment, store it, and use it in response to internal and/or external inputs[7][8]. Although different regions of the brain are involved in inputs and outputs that lead to cognitive responses, cognitive function is supported by the forebrain, in particular by the cortex. Reptiles are able to perform a number of cognitive tasks. These include learning; forming memories; spatial navigation; visual and social learning; performing novel behavior; and consciousness-related cognitive tasks [9][10]. Reptiles are also capable of distinguishing between different quantities, shapes, and colors; can learn to press buttons to receive food rewards and to accurately navigate mazes; and exhibit heterospecific and conspecific social learning[10].
The hindbrain coordinates and integrates sensory (e.g. touch) and motor (e.g. swimming) inputs and outputs from internal and external environments via axons from the spinal cord[4]. The midbrain integrates sensory and motor inputs with behavioral responses from the hindbrain to the forebrain[4]. The forebrain corresponds to complex sensory, locomotor, cognitive, and social behaviors[10][9]. The forebrain, is further divided into diencephalon and telencephalon. Diencephalon is related to regulation of eye and body movement in response to visual stimuli, sensory information, circadian rhythms, olfactory input, and autonomic nervous system.Telencephalon is related to control of movements, presence of neurotransmitters and neuromodulators that integrate inputs and transmit output signals from other parts of the brain, sensory systems, and cognitive functions[4].
The turtle cortex has been functionally correlated to behavioral regulation, cognition, navigation, and memory[10]. Turtles and goldfish, like mammals and birds, can accurately and flexibly navigate towards a goal across direct route using landmarks to encode spatial relationships creating a map-like representation, even when exposed to unvisited start locations[10].The medial and dorsomedial cortex in turtles is homologous to the mammalian dentate gyrus and hippocampus, respectively[11][4]. The dorsal cortex receives visual and somatosensory projections[4]. The lateral cortex is homologous to the olfactory cortex of mammals, also receiving olfactory projections[4]. Another area of the forebrain associated with cognition is the DVR (dorsal ventricular ridge), which is particularly enlarged and cytologically complex in non-avian reptiles, and has been associated with complex social behaviors, visual, auditory, and somatosensory projections[9].
Comparison with Mammalian Brain
[edit]The turtle brain is more similar to the mammalian brain than previously known[10][5][12][11], contrary to the Triune Brain Theory, which stated that the reptilian brain is a simplified version of the mammalian brain, composed solely of the basal ganglia responsible for primal instinct behaviors[11][5][13][14]. In fact, the reptilian brain shares the same excitatory and inhibitory neurons; same physiological properties of pyramidal neurons; sensory inputs; and several neuronal circuits with the mammalian brain[11][5][12][15]. Furthermore, clusters of similar neurons have been found in all regions of the reptilian and mammalian brains and share high levels of the same transcription factors[13].
The reptilian cortex is composed of three-layers of neuronal tissue, while the mammalian cortex has six-layers[5], with the exception of cetaceans which have five layers[14]. The difference in numbers of layers led to the conclusion that the neocortex is a mammalian novelty. However, recent studies have shown that the mammalian cortex has conserved transcription factors found in the common ancestor of reptiles and mammals suggesting the neocortex may not be a novel character[11][13].
To be added to Brain page:
Anatomy
[edit]Evolution
[edit]Reptiles
[edit]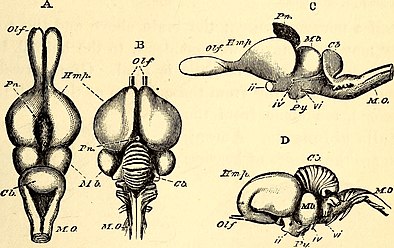

Modern reptiles and mammals diverged from a common ancestor around 320 million years ago[5]. Interestingly, the number of extant reptiles far exceeds the number of mammalian species, with 11,733 recognized species of reptiles[16] compared to 5,884 extant mammals[17]. Along with the species diversity, reptiles have diverged in terms of external morphology, from limbless to tetrapod gliders to armored chelonians, reflecting adaptive radiation to a diverse array of environments[14][10].
Morphological differences are reflected in the nervous system phenotype, such as: absence of lateral motor column neurons in snakes, which innervate limb muscles controlling limb movements; absence of motor neurons that innervate trunk muscles in tortoises; presence of innervation from the trigeminal nerve to pit organs responsible to infrared detection in snakes[14]. Variation in size, weight, and shape of the brain can be found within reptiles[9]. For instance, crocodilians have the largest brain volume to body weight proportion, followed by turtles, lizards, and snakes. Reptiles vary in the investment in different brain sections. Crocodilians have the largest telencephalon, while snakes have the smallest. Turtles have the largest diencephalon per body weight whereas crocodilians have the smallest. On the other hand, lizards have the largest mesencephalon[9].
Yet their brains share several characteristics revealed by recent anatomical, molecular, and ontogenetic studies[11][13][18]. Vertebrates share the highest levels of similarities during embryological development, controlled by conserved transcription factors and signaling centers, including gene expression, morphological and cell type differentiation[11][14][19]. In fact, high levels of transcriptional factors can be found in all areas of the brain in reptiles and mammals, with shared neuronal clusters enlightening brain evolution[13]. Conserved transcription factors elucidate that evolution acted in different areas of the brain by either retaining similar morphology and function, or diversifying it[11][13].
Anatomically, the reptilian brain has less subdivisions than the mammalian brain, however it has numerous conserved aspects including the organization of the spinal cord and cranial nerve, as well as elaborated brain pattern of organization[4]. Elaborated brains are characterized by migrated neuronal cell bodies away from the periventricular matrix, region of neuronal development, forming organized nuclear groups[4]. Aside from reptiles and mammals, other vertebrates with elaborated brains include hagfish, galeomorph sharks, skates, rays, teleosts, and birds[4]. Overall elaborated brains are subdivided in forebrain, midbrain, and hindbrain.
The hindbrain coordinates and integrates sensory and motor inputs and outputs responsible for, but not limited to, walking, swimming, or flying. It contains input and output axons interconnecting the spinal cord, midbrain and forebrain transmitting information from the external and internal environments[4]. The midbrain links sensory, motor, and integrative components received from the hindbrain, connecting it to the forebrain. The tectum, which includes the optic tectum and torus semicircularis, receives auditory, visual, and somatosensory inputs, forming integrated maps of the sensory and visual space around the animal[4]. The tegmentum receives incoming sensory information and forwards motor responses to and from the forebrain. The isthmus connects the hindbrain with midbrain. The forebrain region is particularly well developed, is further divided into diencephalon and telencephalon. Diencephalon is related to regulation of eye and body movement in response to visual stimuli, sensory information, circadian rhythms, olfactory input, and autonomic nervous system.Telencephalon is related to control of movements, neurotransmitters and neuromodulators responsible for integrating inputs and transmitting outputs are present, sensory systems, and cognitive functions[4].
References
[edit]- ^ Wyneken, Jeanette (2007-09). "Reptilian Neurology: Anatomy and Function". Veterinary Clinics of North America: Exotic Animal Practice. 10 (3): 837–853. doi:10.1016/j.cvex.2007.05.004. ISSN 1094-9194.
{{cite journal}}
: Check date values in:|date=
(help) - ^ a b M., Ashley, Laurence (1980). Laboratory anatomy of the turtle. W.C. Brown. ISBN 0-697-04601-X. OCLC 757230160.
{{cite book}}
: CS1 maint: multiple names: authors list (link) - ^ a b Hall, David H. (1995). An Illustrated Dissection Guide to the Turtle. Tucson, Arizona: Ranaco.
- ^ a b c d e f g h i j k l m n o William, Butler, Ann B. Hodos, (2005). Comparative vertebrate neuroanatomy : evolution and adaptation. Wiley-Liss. ISBN 0-471-21005-6. OCLC 489018202.
{{cite book}}
: CS1 maint: extra punctuation (link) CS1 maint: multiple names: authors list (link) - ^ a b c d e f g Reiter, Sam; Liaw, Hua-Peng; Yamawaki, Tracy M.; Naumann, Robert K.; Laurent, Gilles (2017). "On the Value of Reptilian Brains to Map the Evolution of the Hippocampal Formation". Brain, Behavior and Evolution. 90 (1): 41–52. doi:10.1159/000478693. ISSN 0006-8977. PMID 28866680.
- ^ Shen, J. M.; Kriegstein, A. R. (1986-12-01). "Turtle hippocampal cortex contains distinct cell types, burst-firing neurons, and an epileptogenic subfield". Journal of Neurophysiology. 56 (6): 1626–1649. doi:10.1152/jn.1986.56.6.1626. ISSN 0022-3077.
- ^ Roth, Timothy C.; Krochmal, Aaron R.; LaDage, Lara D. (2019-08). "Reptilian Cognition: A More Complex Picture via Integration of Neurological Mechanisms, Behavioral Constraints, and Evolutionary Context". BioEssays. 41 (8): 1900033. doi:10.1002/bies.201900033. ISSN 0265-9247.
{{cite journal}}
: Check date values in:|date=
(help) - ^ Szabo, Birgit; Noble, Daniel W. A.; Whiting, Martin J. (2021-04). "Learning in non‐avian reptiles 40 years on: advances and promising new directions". Biological Reviews. 96 (2): 331–356. doi:10.1111/brv.12658. ISSN 1464-7931.
{{cite journal}}
: Check date values in:|date=
(help) - ^ a b c d e Northcutt, R. Glenn (2013). "Variation in Reptilian Brains and Cognition". Brain, Behavior and Evolution. 82 (1): 45–54. doi:10.1159/000351996. ISSN 0006-8977. PMID 23979455.
- ^ a b c d e f g Salas, Cosme; Broglio, Cristina; Rodríguez, Fernando (2003). "Evolution of Forebrain and Spatial Cognition in Vertebrates: Conservation across Diversity". Brain, Behavior and Evolution. 62 (2): 72–82. doi:10.1159/000072438. ISSN 0006-8977. PMID 12937346.
- ^ a b c d e f g h Naumann, Robert K.; Ondracek, Janie M.; Reiter, Samuel; Shein-Idelson, Mark; Tosches, Maria Antonietta; Yamawaki, Tracy M.; Laurent, Gilles (2015-04-20). "The reptilian brain". Current Biology. 25 (8): R317–R321. doi:10.1016/j.cub.2015.02.049. ISSN 0960-9822. PMC 4406946. PMID 25898097.
{{cite journal}}
: CS1 maint: PMC format (link) - ^ a b Blanton, Mark G.; Kriegstein, Arnold R. (1991-08-22). "Morphological differentiation of distinct neuronal classes in embryonic turtle cerebral cortex". The Journal of Comparative Neurology. 310 (4): 550–570. doi:10.1002/cne.903100405. ISSN 0021-9967.
- ^ a b c d e f Hain, David; Gallego-Flores, Tatiana; Klinkmann, Michaela; Macias, Angeles; Ciirdaeva, Elena; Arends, Anja; Thum, Christina; Tushev, Georgi; Kretschmer, Friedrich; Tosches, Maria Antonietta; Laurent, Gilles (2022-09-02). "Molecular diversity and evolution of neuron types in the amniote brain". Science. 377 (6610): eabp8202. doi:10.1126/science.abp8202. ISSN 0036-8075.
- ^ a b c d e Nomura, Tadashi; Kawaguchi, Masahumi; Ono, Katsuhiko; Murakami, Yasunori (2013-03). "Reptiles: A New Model for Brain Evo-Devo Research: REPTILES FOR EVO-DEVO RESEARCH". Journal of Experimental Zoology Part B: Molecular and Developmental Evolution. 320 (2): 57–73. doi:10.1002/jez.b.22484.
{{cite journal}}
: Check date values in:|date=
(help) - ^ Larkum, Matthew E.; Watanabe, Shigeo; Lasser-Ross, Nechama; Rhodes, Paul; Ross, William N. (2008-02). "Dendritic Properties of Turtle Pyramidal Neurons". Journal of Neurophysiology. 99 (2): 683–694. doi:10.1152/jn.01076.2007. ISSN 0022-3077.
{{cite journal}}
: Check date values in:|date=
(help) - ^ "Species Statistics Aug 2019". www.reptile-database.org. Retrieved 2022-12-06.
- ^ "The IUCN Red List of Threatened Species. Version 2022-1 - Summary Statistics". IUCN Red List. 2022. ISSN 2307-8235. Retrieved December 06, 2022.
{{cite web}}
: Check date values in:|access-date=
(help)CS1 maint: date and year (link) CS1 maint: url-status (link) - ^ Tosches, Maria Antonietta; Yamawaki, Tracy M.; Naumann, Robert K.; Jacobi, Ariel A.; Tushev, Georgi; Laurent, Gilles (2018-05-25). "Evolution of pallium, hippocampus, and cortical cell types revealed by single-cell transcriptomics in reptiles". Science. 360 (6391): 881–888. doi:10.1126/science.aar4237. ISSN 0036-8075.
- ^ Blanton, Mark G.; Kriegstein, Arnold R. (1991-08-22). "Morphological differentiation of distinct neuronal classes in embryonic turtle cerebral cortex". The Journal of Comparative Neurology. 310 (4): 550–570. doi:10.1002/cne.903100405. ISSN 0021-9967.