Stellar kinematics

In astronomy, stellar kinematics is the observational study or measurement of the kinematics or motions of stars through space.
Stellar kinematics encompasses the measurement of stellar velocities in the Milky Way and its satellites as well as the internal kinematics of more distant galaxies. Measurement of the kinematics of stars in different subcomponents of the Milky Way including the thin disk, the thick disk, the bulge, and the stellar halo provides important information about the formation and evolutionary history of our Galaxy. Kinematic measurements can also identify exotic phenomena such as hypervelocity stars escaping from the Milky Way, which are interpreted as the result of gravitational encounters of binary stars with the supermassive black hole at the Galactic Center.
Stellar kinematics is related to but distinct from the subject of stellar dynamics, which involves the theoretical study or modeling of the motions of stars under the influence of gravity. Stellar-dynamical models of systems such as galaxies or star clusters are often compared with or tested against stellar-kinematic data to study their evolutionary history and mass distributions, and to detect the presence of dark matter or supermassive black holes through their gravitational influence on stellar orbits.
Space velocity
[edit]The component of stellar motion toward or away from the Sun, known as radial velocity, can be measured from the spectrum shift caused by the Doppler effect. The transverse, or proper motion must be found by taking a series of positional determinations against more distant objects. Once the distance to a star is determined through astrometric means such as parallax, the space velocity can be computed.[2] This is the star's actual motion relative to the Sun or the local standard of rest (LSR). The latter is typically taken as a position at the Sun's present location that is following a circular orbit around the Galactic Center at the mean velocity of those nearby stars with low velocity dispersion.[3] The Sun's motion with respect to the LSR is called the "peculiar solar motion".
The components of space velocity in the Milky Way's Galactic coordinate system are usually designated U, V, and W, given in km/s, with U positive in the direction of the Galactic Center, V positive in the direction of galactic rotation, and W positive in the direction of the North Galactic Pole.[4] The peculiar motion of the Sun with respect to the LSR is[5]
- (U, V, W) = (11.1, 12.24, 7.25) km/s,
with statistical uncertainty (+0.69−0.75, +0.47−0.47, +0.37−0.36) km/s and systematic uncertainty (1, 2, 0.5) km/s. (Note that V is 7 km/s larger than estimated in 1998 by Dehnen et al.[6])
Use of kinematic measurements
[edit]Stellar kinematics yields important astrophysical information about stars, and the galaxies in which they reside. Stellar kinematics data combined with astrophysical modeling produces important information about the galactic system as a whole. Measured stellar velocities in the innermost regions of galaxies including the Milky Way have provided evidence that many galaxies host supermassive black holes at their center. In farther out regions of galaxies such as within the galactic halo, velocity measurements of globular clusters orbiting in these halo regions of galaxies provides evidence for dark matter. Both of these cases derive from the key fact that stellar kinematics can be related to the overall potential in which the stars are bound. This means that if accurate stellar kinematics measurements are made for a star or group of stars orbiting in a certain region of a galaxy, the gravitational potential and mass distribution can be inferred given that the gravitational potential in which the star is bound produces its orbit and serves as the impetus for its stellar motion. Examples of using kinematics combined with modeling to construct an astrophysical system include:
- Rotation of the Milky Way's disc: From the proper motions and radial velocities of stars within the Milky way disc one can show that there is differential rotation. When combining these measurements of stars' proper motions and their radial velocities, along with careful modeling, it is possible to obtain a picture of the rotation of the Milky Way disc. The local character of galactic rotation in the solar neighborhood is encapsulated in the Oort constants.[7][8][9]
- Structural components of the Milky Way: Using stellar kinematics, astronomers construct models which seek to explain the overall galactic structure in terms of distinct kinematic populations of stars. This is possible because these distinct populations are often located in specific regions of galaxies. For example, within the Milky Way, there are three primary components, each with its own distinct stellar kinematics: the disc, halo and bulge or bar. These kinematic groups are closely related to the stellar populations in the Milky Way, forming a strong correlation between the motion and chemical composition, thus indicating different formation mechanisms. For the Milky Way, the speed of disk stars is and an RMS (Root mean square) velocity relative to this speed of . For bulge population stars, the velocities are randomly oriented with a larger relative RMS velocity of and no net circular velocity.[10] The Galactic stellar halo consists of stars with orbits that extend to the outer regions of the galaxy. Some of these stars will continually orbit far from the galactic center, while others are on trajectories which bring them to various distances from the galactic center. These stars have little to no average rotation. Many stars in this group belong to globular clusters which formed long ago and thus have a distinct formation history, which can be inferred from their kinematics and poor metallicities. The halo may be further subdivided into an inner and outer halo, with the inner halo having a net prograde motion with respect to the Milky Way and the outer a net retrograde motion.[11]
- External galaxies: Spectroscopic observations of external galaxies make it possible to characterize the bulk motions of the stars they contain. While these stellar populations in external galaxies are generally not resolved to the level where one can track the motion of individual stars (except for the very nearest galaxies) measurements of the kinematics of the integrated stellar population along the line of sight provides information including the mean velocity and the velocity dispersion which can then be used to infer the distribution of mass within the galaxy. Measurement of the mean velocity as a function of position gives information on the galaxy's rotation, with distinct regions of the galaxy that are redshifted / blueshifted in relation to the galaxy's systemic velocity.
- Mass distributions: Through measurement of the kinematics of tracer objects such as globular clusters and the orbits of nearby satellite dwarf galaxies, we can determine the mass distribution of the Milky Way or other galaxies. This is accomplished by combining kinematic measurements with dynamical modeling.
Recent advancements due to Gaia
[edit]
In 2018, the Gaia Data Release 2 (GAIA DR2) marked a significant advancement in stellar kinematics, offering a rich dataset of precise measurements. This release included detailed stellar kinematic and stellar parallax data, contributing to a more nuanced understanding of the Milky Way's structure. Notably, it facilitated the determination of proper motions for numerous celestial objects, including the absolute proper motions of 75 globular clusters situated at distances extending up to and a bright limit of .[12] Furthermore, Gaia's comprehensive dataset enabled the measurement of absolute proper motions in nearby dwarf spheroidal galaxies, serving as crucial indicators for understanding the mass distribution within the Milky Way.[13] GAIA DR3 improved the quality of previously published data by providing detailed astrophysical parameters.[14] While the complete GAIA DR4 is yet to be unveiled, the latest release offers enhanced insights into white dwarfs, hypervelocity stars, cosmological gravitational lensing, and the merger history of the Galaxy.[15]
Stellar kinematic types
[edit]Stars within galaxies may be classified based on their kinematics. For example, the stars in the Milky Way can be subdivided into two general populations, based on their metallicity, or proportion of elements with atomic numbers higher than helium. Among nearby stars, it has been found that population I stars with higher metallicity are generally located in the stellar disk while older population II stars are in random orbits with little net rotation.[16] The latter have elliptical orbits that are inclined to the plane of the Milky Way.[16] Comparison of the kinematics of nearby stars has also led to the identification of stellar associations. These are most likely groups of stars that share a common point of origin in giant molecular clouds.[17]
There are many additional ways to classify stars based on their measured velocity components, and this provides detailed information about the nature of the star's formation time, its present location, and the general structure of the galaxy. As a star moves in a galaxy, the smoothed out gravitational potential of all the other stars and other mass within the galaxy plays a dominant role in determining the stellar motion.[18] Stellar kinematics can provide insights into the location of where the star formed within the galaxy. Measurements of an individual star's kinematics can identify stars that are peculiar outliers such as a high-velocity star moving much faster than its nearby neighbors.
High-velocity stars
[edit]Depending on the definition, a high-velocity star is a star moving faster than 65 km/s to 100 km/s relative to the average motion of the other stars in the star's neighborhood. The velocity is also sometimes defined as supersonic relative to the surrounding interstellar medium. The three types of high-velocity stars are: runaway stars, halo stars and hypervelocity stars. High-velocity stars were studied by Jan Oort, who used their kinematic data to predict that high-velocity stars have very little tangential velocity.[19]
Runaway stars
[edit]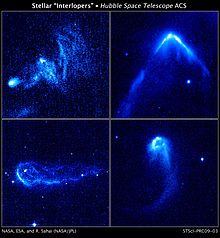
A runaway star is one that is moving through space with an abnormally high velocity relative to the surrounding interstellar medium. The proper motion of a runaway star often points exactly away from a stellar association, of which the star was formerly a member, before it was hurled out.
Mechanisms that may give rise to a runaway star include:
- Gravitational interactions between stars in a stellar system can result in large accelerations of one or more of the involved stars. In some cases, stars may even be ejected.[20] This can occur in seemingly stable star systems of only three stars, as described in studies of the three-body problem in gravitational theory.[21]
- A collision or close encounter between stellar systems, including galaxies, may result in the disruption of both systems, with some of the stars being accelerated to high velocities, or even ejected. A large-scale example is the gravitational interaction between the Milky Way and the Large Magellanic Cloud.[22]
- A supernova explosion in a multiple star system can accelerate both the supernova remnant and remaining stars to high velocities.[23][24]
Multiple mechanisms may accelerate the same runaway star. For example, a massive star that was originally ejected due to gravitational interactions with its stellar neighbors may itself go supernova, producing a remnant with a velocity modulated by the supernova kick. If this supernova occurs in the very nearby vicinity of other stars, it is possible that it may produce more runaways in the process.
An example of a related set of runaway stars is the case of AE Aurigae, 53 Arietis and Mu Columbae, all of which are moving away from each other at velocities of over 100 km/s (for comparison, the Sun moves through the Milky Way at about 20 km/s faster than the local average). Tracing their motions back, their paths intersect near to the Orion Nebula about 2 million years ago. Barnard's Loop is believed to be the remnant of the supernova that launched the other stars.
Another example is the X-ray object Vela X-1, where photodigital techniques reveal the presence of a typical supersonic bow shock hyperbola.
Halo stars
[edit]Halo stars are very old stars that do not follow circular orbits around the center of the Milky Way within its disk. Instead, the halo stars travel in elliptical orbits, often inclined to the disk, which take them well above and below the plane of the Milky Way. Although their orbital velocities relative to the Milky Way may be no faster than disk stars, their different paths result in high relative velocities.
Typical examples are the halo stars passing through the disk of the Milky Way at steep angles. One of the nearest 45 stars, called Kapteyn's Star, is an example of the high-velocity stars that lie near the Sun: Its observed radial velocity is −245 km/s, and the components of its space velocity are u = +19 km/s, v = −288 km/s, and w = −52 km/s.
Hypervelocity stars
[edit]
Hypervelocity stars (designated as HVS or HV in stellar catalogues) have substantially higher velocities than the rest of the stellar population of a galaxy. Some of these stars may even exceed the escape velocity of the galaxy.[25] In the Milky Way, stars usually have velocities on the order of 100 km/s, whereas hypervelocity stars typically have velocities on the order of 1000 km/s. Most of these fast-moving stars are thought to be produced near the center of the Milky Way, where there is a larger population of these objects than further out. One of the fastest known stars in our Galaxy is the O-class sub-dwarf US 708, which is moving away from the Milky Way with a total velocity of around 1200 km/s.
Jack G. Hills first predicted the existence of HVSs in 1988.[26] This was later confirmed in 2005 by Warren Brown, Margaret Geller, Scott Kenyon, and Michael Kurtz.[27] As of 2008,[update] 10 unbound HVSs were known, one of which is believed to have originated from the Large Magellanic Cloud rather than the Milky Way.[28] Further measurements placed its origin within the Milky Way.[29] Due to uncertainty about the distribution of mass within the Milky Way, determining whether a HVS is unbound is difficult. A further five known high-velocity stars may be unbound from the Milky Way, and 16 HVSs are thought to be bound. The nearest currently known HVS (HVS2) is about 19 kpc from the Sun.
As of 1 September 2017[update], there have been roughly 20 observed hypervelocity stars. Though most of these were observed in the Northern Hemisphere, the possibility remains that there are HVSs only observable from the Southern Hemisphere.[30]
It is believed that about 1,000 HVSs exist in the Milky Way.[31] Considering that there are around 100 billion stars in the Milky Way, this is a minuscule fraction (~0.000001%). Results from the second data release of Gaia (DR2) show that most high-velocity late-type stars have a high probability of being bound to the Milky Way.[32] However, distant hypervelocity star candidates are more promising.[33]
In March 2019, LAMOST-HVS1 was reported to be a confirmed hypervelocity star ejected from the stellar disk of the Milky Way.[34]
In July 2019, astronomers reported finding an A-type star, S5-HVS1, traveling 1,755 km/s (3,930,000 mph), faster than any other star detected so far. The star is in the Grus (or Crane) constellation in the southern sky and is about 29,000 ly (1.8×109 AU) from Earth. It may have been ejected from the Milky Way after interacting with Sagittarius A*, the supermassive black hole at the center of the galaxy.[35][36][37][38][39]
Origin of hypervelocity stars
[edit]
HVSs are believed to predominantly originate by close encounters of binary stars with the supermassive black hole in the center of the Milky Way. One of the two partners is gravitationally captured by the black hole (in the sense of entering orbit around it), while the other escapes with high velocity, becoming a HVS. Such maneuvers are analogous to the capture and ejection of interstellar objects by a star.
Supernova-induced HVSs may also be possible, although they are presumably rare. In this scenario, a HVS is ejected from a close binary system as a result of the companion star undergoing a supernova explosion. Ejection velocities up to 770 km/s, as measured from the galactic rest frame, are possible for late-type B-stars.[40] This mechanism can explain the origin of HVSs which are ejected from the galactic disk.
Known HVSs are main-sequence stars with masses a few times that of the Sun. HVSs with smaller masses are also expected and G/K-dwarf HVS candidates have been found.
Some HVSs may have originated from a disrupted dwarf galaxy. When it made its closest approach to the center of the Milky Way, some of its stars broke free and were thrown into space, due to the slingshot-like effect of the boost.[41]
Some neutron stars are inferred to be traveling with similar speeds. This could be related to HVSs and the HVS ejection mechanism. Neutron stars are the remnants of supernova explosions, and their extreme speeds are very likely the result of an asymmetric supernova explosion or the loss of their near partner during the supernova explosions that forms them. The neutron star RX J0822-4300, which was measured to move at a record speed of over 1,500 km/s (0.5% of the speed of light) in 2007 by the Chandra X-ray Observatory, is thought to have been produced the first way.[42]
One theory regarding the ignition of Type Ia supernovae invokes the onset of a merger between two white dwarfs in a binary star system, triggering the explosion of the more massive white dwarf. If the less massive white dwarf is not destroyed during the explosion, it will no longer be gravitationally bound to its destroyed companion, causing it to leave the system as a hypervelocity star with its pre-explosion orbital velocity of 1000–2500 km/s. In 2018, three such stars were discovered using data from the Gaia satellite.[43]
Partial list of HVSs
[edit]As of 2014, twenty HVS were known.[44][31]
- HVS 1 – (SDSS J090744.99+024506.8) (a.k.a. The Outcast Star) – the first hypervelocity star to be discovered[27]
- HVS 2 – (SDSS J093320.86+441705.4 or US 708)
- HVS 3 – (HE 0437-5439) – possibly from the Large Magellanic Cloud[28]
- HVS 4 – (SDSS J091301.00+305120.0)
- HVS 5 – (SDSS J091759.42+672238.7)
- HVS 6 – (SDSS J110557.45+093439.5)
- HVS 7 – (SDSS J113312.12+010824.9)
- HVS 8 – (SDSS J094214.04+200322.1)
- HVS 9 – (SDSS J102137.08-005234.8)
- HVS 10 – (SDSS J120337.85+180250.4)
Kinematic groups
[edit]A set of stars with similar space motion and ages is known as a kinematic group.[45] These are stars that could share a common origin, such as the evaporation of an open cluster, the remains of a star forming region, or collections of overlapping star formation bursts at differing time periods in adjacent regions.[46] Most stars are born within molecular clouds known as stellar nurseries. The stars formed within such a cloud compose gravitationally bound open clusters containing dozens to thousands of members with similar ages and compositions. These clusters dissociate with time. Groups of young stars that escape a cluster, or are no longer bound to each other, form stellar associations. As these stars age and disperse, their association is no longer readily apparent and they become moving groups of stars.
Astronomers are able to determine if stars are members of a kinematic group because they share the same age, metallicity, and kinematics (radial velocity and proper motion). As the stars in a moving group formed in proximity and at nearly the same time from the same gas cloud, although later disrupted by tidal forces, they share similar characteristics.[47]
Stellar associations
[edit]A stellar association is a very loose star cluster, whose stars share a common origin and are still moving together through space, but have become gravitationally unbound. Associations are primarily identified by their common movement vectors and ages. Identification by chemical composition is also used to factor in association memberships.
Stellar associations were first discovered by the Armenian astronomer Viktor Ambartsumian in 1947.[48] The conventional name for an association uses the names or abbreviations of the constellation (or constellations) in which they are located; the association type, and, sometimes, a numerical identifier.
Types
[edit]
Viktor Ambartsumian first categorized stellar associations into two groups, OB and T, based on the properties of their stars.[48] A third category, R, was later suggested by Sidney van den Bergh for associations that illuminate reflection nebulae.[49] The OB, T, and R associations form a continuum of young stellar groupings. But it is currently uncertain whether they are an evolutionary sequence, or represent some other factor at work.[50] Some groups also display properties of both OB and T associations, so the categorization is not always clear-cut.
OB associations
[edit]
Young associations will contain 10 to 100 massive stars of spectral class O and B, and are known as OB associations. In addition, these associations also contain hundreds or thousands of low- and intermediate-mass stars. Association members are believed to form within the same small volume inside a giant molecular cloud. Once the surrounding dust and gas is blown away, the remaining stars become unbound and begin to drift apart.[51] It is believed that the majority of all stars in the Milky Way were formed in OB associations.[51] O-class stars are short-lived, and will expire as supernovae after roughly one million years. As a result, OB associations are generally only a few million years in age or less. The O-B stars in the association will have burned all their fuel within ten million years. (Compare this to the current age of the Sun at about five billion years.)
The Hipparcos satellite provided measurements that located a dozen OB associations within 650 parsecs of the Sun.[52] The nearest OB association is the Scorpius–Centaurus association, located about 400 light-years from the Sun.[53]
OB associations have also been found in the Large Magellanic Cloud and the Andromeda Galaxy. These associations can be quite sparse, spanning 1,500 light-years in diameter.[17]
T associations
[edit]Young stellar groups can contain a number of infant T Tauri stars that are still in the process of entering the main sequence. These sparse populations of up to a thousand T Tauri stars are known as T associations. The nearest example is the Taurus-Auriga T association (Tau–Aur T association), located at a distance of 140 parsecs from the Sun.[54] Other examples of T associations include the R Corona Australis T association, the Lupus T association, the Chamaeleon T association and the Velorum T association. T associations are often found in the vicinity of the molecular cloud from which they formed. Some, but not all, include O–B class stars. Group members have the same age and origin, the same chemical composition, and the same amplitude and direction in their vector of velocity.
R associations
[edit]Associations of stars that illuminate reflection nebulae are called R associations, a name suggested by Sidney van den Bergh after he discovered that the stars in these nebulae had a non-uniform distribution.[49] These young stellar groupings contain main sequence stars that are not sufficiently massive to disperse the interstellar clouds in which they formed.[50] This allows the properties of the surrounding dark cloud to be examined by astronomers. Because R associations are more plentiful than OB associations, they can be used to trace out the structure of the galactic spiral arms.[55] An example of an R association is Monoceros R2, located 830 ± 50 parsecs from the Sun.[50]
Moving groups
[edit]
If the remnants of a stellar association drift through the Milky Way as a somewhat coherent assemblage, then they are termed a moving group or kinematic group. Moving groups can be old, such as the HR 1614 moving group at two billion years, or young, such as the AB Dor Moving Group at only 120 million years.
Moving groups were studied intensely by Olin Eggen in the 1960s.[56] A list of the nearest young moving groups has been compiled by López-Santiago et al.[45] The closest is the Ursa Major Moving Group which includes all of the stars in the Plough / Big Dipper asterism except for Dubhe and η Ursae Majoris. This is sufficiently close that the Sun lies in its outer fringes, without being part of the group. Hence, although members are concentrated at declinations near 60°N, some outliers are as far away across the sky as Triangulum Australe at 70°S.
The list of young moving groups is constantly evolving. The Banyan Σ tool[57] currently lists 29 nearby young moving groups[59][58] Recent additions to nearby moving groups are the Volans-Carina Association (VCA), discovered with Gaia,[60] and the Argus Association (ARG), confirmed with Gaia.[61] Moving groups can sometimes be further subdivided in smaller distinct groups. The Great Austral Young Association (GAYA) complex was found to be subdivided into the moving groups Carina, Columba, and Tucana-Horologium. The three Associations are not very distinct from each other, and have similar kinematic properties.[62]
Young moving groups have well known ages and can help with the characterization of objects with hard-to-estimate ages, such as brown dwarfs.[63] Members of nearby young moving groups are also candidates for directly imaged protoplanetary disks, such as TW Hydrae or directly imaged exoplanets, such as Beta Pictoris b or GU Psc b.
Stellar streams
[edit]A stellar stream is an association of stars orbiting a galaxy that was once a globular cluster or dwarf galaxy that has now been torn apart and stretched out along its orbit by tidal forces.[64]
Known kinematic groups
[edit]Some nearby kinematic groups include:[45]
- Local Association (Pleiades moving group)
- AB Doradus moving group
- Alpha Persei moving cluster
- Beta Pictoris moving group[65]
- Castor moving group
- Corona Australis association
- Eta Chamaeleontis cluster
- Hercules-Lyra association[45]
- Hercules stream
- Hyades Stream
- IC 2391 supercluster (Argus Association)
- Kapteyn group[66]
- MBM 12 association
- TW Hydrae association[65]
- Ursa Major Moving Group
- Wolf 630 moving group[67]
- Zeta Herculis moving group
- Pisces-Eridanus stellar stream
- Tucana-Horologium association[65]
See also
[edit]- Astrometry
- Gaia (spacecraft)
- Hipparcos
- n-body problem
- Open cluster remnant
- List of nearby stellar associations and moving groups
- Stellar association
References
[edit]- ^ Kaler, James B. (November 2005). "Barnard's Star (V2500 Ophiuchi)". Stars. James B. Kaler. Archived from the original on 5 September 2006. Retrieved 12 July 2018.
- ^ "Stellar Motions (Extension)". Australia Telescope Outreach and Education. Commonwealth Scientific and Industrial Research Organisation. 2005-08-18. Archived from the original on 2013-06-06. Retrieved 2008-11-19.
- ^ Fich, Michel; Tremaine, Scott (1991). "The mass of the Galaxy". Annual Review of Astronomy and Astrophysics. 29 (1): 409–445. Bibcode:1991ARA&A..29..409F. doi:10.1146/annurev.aa.29.090191.002205.
- ^ Johnson, Dean R. H.; Soderblom, David R. (1987). "Calculating galactic space velocities and their uncertainties, with an application to the Ursa Major group". Astronomical Journal. 93 (2): 864–867. Bibcode:1987AJ.....93..864J. doi:10.1086/114370.
- ^ Schönrich, Ralph; Binney, James; Dehnen, Walter (2010). "Local kinematics and the local standard of rest". Monthly Notices of the Royal Astronomical Society. 403 (4): 1829–1833. arXiv:0912.3693. Bibcode:2010MNRAS.403.1829S. doi:10.1111/j.1365-2966.2010.16253.x. S2CID 118697588.
- ^ Dehnen, Walter; Binney, James J. (1998). "Local stellar kinematics from HIPPARCOS data". Monthly Notices of the Royal Astronomical Society. 298 (2): 387–394. arXiv:astro-ph/9710077. Bibcode:1998MNRAS.298..387D. doi:10.1046/j.1365-8711.1998.01600.x. S2CID 15936627.
- ^ Oort, JH (1927). "Observational evidence confirming Lindblad's hypothesis of a rotation of the galactic system". Bulletin of the Astronomical Institutes of the Netherlands. 3: 275–282. Bibcode:1927BAN.....3..275O.
- ^ Li, C; Zhao, G; Yang, C (2019). "Galactic Rotation and the Oort Constants in the Solar Vicinity". The Astrophysical Journal. 872 (2): 205. Bibcode:2019ApJ...872..205L. doi:10.3847/1538-4357/ab0104. S2CID 127759240.
- ^ Olling, RP; Merrifield, MR (1998). "Refining the Oort and Galactic constants". Monthly Notices of the Royal Astronomical Society. 297 (3): 943–952. arXiv:astro-ph/9802034. Bibcode:1998MNRAS.297..943O. doi:10.1046/j.1365-8711.1998.01577.x.
- ^ Binney, James; Tremaine, Scott (2008). Galactic Dynamics. Princeton University Press. pp. 16–19. ISBN 9780691130279.
- ^ Carollo, Daniela; et al. (2007). "Two Stellar Components in the Halo of the Milky Way". Nature. 450 (7172): 1020–1025. arXiv:0706.3005. Bibcode:2007Natur.450.1020C. doi:10.1038/nature06460. PMID 18075581. S2CID 4387133.
- ^ "Gaia DR2 contents – Gaia – Cosmos". www.cosmos.esa.int. Retrieved 2024-03-08.
- ^ Watkins, Laura; et al. (May 2018). "Evidence for an Intermediate-Mass Milky Way from Gaia DR2 Halo Globular Cluster Motions". The Astrophysical Journal. 873 (2): 118. arXiv:1804.11348. Bibcode:2019ApJ...873..118W. doi:10.3847/1538-4357/ab089f. S2CID 85463973.
- ^ Fouesneau, M.; Frémat, Y.; Andrae, R.; Korn, A. J.; Soubiran, C.; Kordopatis, G.; Vallenari, A.; Heiter, U.; Creevey, O. L.; Sarro, L. M.; Laverny, P. de; Lanzafame, A. C.; Lobel, A.; Sordo, R.; Rybizki, J. (2023-06-01). "Gaia Data Release 3 – Apsis. II. Stellar parameters". Astronomy & Astrophysics. 674: A28. arXiv:2206.05992. Bibcode:2023A&A...674A..28F. doi:10.1051/0004-6361/202243919. ISSN 0004-6361.
- ^ Hodgkin, S. T.; Harrison, D. L.; Breedt, E.; Wevers, T.; Rixon, G.; Delgado, A.; Yoldas, A.; Kostrzewa-Rutkowska, Z.; Wyrzykowski, Ł; Leeuwen, M. van; Blagorodnova, N.; Campbell, H.; Eappachen, D.; Fraser, M.; Ihanec, N. (2021-08-01). "Gaia Early Data Release 3 - Gaia photometric science alerts". Astronomy & Astrophysics. 652: A76. arXiv:2106.01394. Bibcode:2021A&A...652A..76H. doi:10.1051/0004-6361/202140735. ISSN 0004-6361.
- ^ a b Johnson, Hugh M. (1957). "The Kinematics and Evolution of Population I Stars". Publications of the Astronomical Society of the Pacific. 69 (406): 54. Bibcode:1957PASP...69...54J. doi:10.1086/127012.
- ^ a b Elmegreen, B.; Nikolaevich Efremov, Y. (1998). "The Formation of Star Clusters". American Scientist. 86 (3): 264. Bibcode:1998AmSci..86..264E. doi:10.1511/1998.3.264. S2CID 262334560. Archived from the original on 2016-07-01. Retrieved 2006-08-23.
- ^ Sparke, L. S.; Gallagher, J. S. (2007). Galaxies in the Universe. United States: Cambridge University Press. p. 111. ISBN 978-0521671866.
- ^ Binney, James; Merrifield, Michael (1998). Galactic Astronomy. Princeton University Press. pp. 16–17. ISBN 978-0691004020.
- ^ Oh, Seungkyung; Kroupa, Pavel; Pflamm-Altenburg, Jan (2015). "Dependency of Dynamical Ejections of O Stars on the Masses of Very Young Star Clusters". The Astrophysical Journal. 805 (2): 92. arXiv:1503.08827. Bibcode:2015ApJ...805...92O. doi:10.1088/0004-637X/805/2/92. ISSN 0004-637X. S2CID 119255350.
- ^ Gvaramadze, Vasilii V.; Gualandris, Alessia (2010-09-30). "Very massive runaway stars from three-body encounters". Monthly Notices of the Royal Astronomical Society. 410 (1): 304–312. arXiv:1007.5057. Bibcode:2011MNRAS.410..304G. doi:10.1111/j.1365-2966.2010.17446.x. ISSN 0035-8711. S2CID 123481910.
- ^ Boubert, D.; Erkal, D.; Evans, N. W.; Izzard, R. G. (2017-04-10). "Hypervelocity runaways from the Large Magellanic Cloud". Monthly Notices of the Royal Astronomical Society. 469 (2): 2151–2162. arXiv:1704.01373. Bibcode:2017MNRAS.469.2151B. doi:10.1093/mnras/stx848. ISSN 0035-8711.
- ^ Blaauw, A. (1961). "On the origin of the O- and B-type stars with high velocities (the run-away stars), and some related problems". Bulletin of the Astronomical Institutes of the Netherlands. 15: 265. Bibcode:1961BAN....15..265B.
- ^ Tauris, T.M.; Takens, R.J. (1998). "Runaway velocities of stellar components originating from disrupted binaries via asymmetric supernova explosions". Astronomy and Astrophysics. 330: 1047–1059. Bibcode:1998A&A...330.1047T.
- ^ "Two Exiled Stars Are Leaving Our Galaxy Forever". Space Daily. Jan 27, 2006. Retrieved 2009-09-24.
- ^ Hills, J. G. (1988). "Hyper-velocity and tidal stars from binaries disrupted by a massive Galactic black hole". Nature. 331 (6158): 687–689. Bibcode:1988Natur.331..687H. doi:10.1038/331687a0. S2CID 4250308.
- ^ a b Brown, Warren R.; Geller, Margaret J.; Kenyon, Scott J.; Kurtz, Michael J. (2005). "Discovery of an Unbound Hypervelocity Star in the Milky Way Halo". The Astrophysical Journal. 622 (1): L33–L36. arXiv:astro-ph/0501177. Bibcode:2005ApJ...622L..33B. doi:10.1086/429378. S2CID 14322324.
- ^ a b Edelmann, H.; Napiwotzki, R.; Heber, U.; Christlieb, N.; et al. (2005). "HE 0437-5439: An Unbound Hypervelocity Main-Sequence B-Type Star". The Astrophysical Journal. 634 (2): L181–L184. arXiv:astro-ph/0511321. Bibcode:2005ApJ...634L.181E. doi:10.1086/498940. S2CID 15189914.
- ^ Brown, Warren R.; Anderson, Jay; Gnedin, Oleg Y.; Bond, Howard E.; et al. (July 19, 2010). "A Galactic Origin For HE 0437–5439, The Hypervelocity Star Near The Large Magellanic Cloud". The Astrophysical Journal Letters. 719 (1): L23. arXiv:1007.3493. Bibcode:2010ApJ...719L..23B. doi:10.1088/2041-8205/719/1/L23. S2CID 55832434.
- ^ "The Milky Way's fastest stars are runaways". Science and Children: 14. 1 Sep 2017. Retrieved 11 Feb 2018.
- ^ a b Brown, Warren R.; Geller, Margaret J.; Kenyon, Scott J.; Kurtz, Michael J.; Bromley, Benjamin C. (2007). "Hypervelocity Stars. III. The Space Density and Ejection History of Main-Sequence Stars from the Galactic Center". The Astrophysical Journal. 671 (2): 1708–1716. arXiv:0709.1471. Bibcode:2007ApJ...671.1708B. doi:10.1086/523642. S2CID 15074398.
- ^ Boubert, Douglas; Guillochon, James; Hawkins, Keith; Ginsburg, Idan; Evans, N. Wyn; Strader, Jay (6 June 2018). "Revisiting hypervelocity stars after Gaia DR2". Monthly Notices of the Royal Astronomical Society. 479 (2): 2789–2795. arXiv:1804.10179. Bibcode:2018MNRAS.479.2789B. doi:10.1093/mnras/sty1601.
- ^ de la Fuente Marcos, R.; de la Fuente Marcos, C. (8 July 2019). "Flying far and fast: the distribution of distant hypervelocity star candidates from Gaia DR2 data". Astronomy and Astrophysics. 627: A104 (17 pp.). arXiv:1906.05227. Bibcode:2019A&A...627A.104D. doi:10.1051/0004-6361/201935008. S2CID 186207054.
- ^ University of Michigan (13 March 2019). "Researchers confirm massive hyper-runaway star ejected from the Milky Way Disk". Phys.org. Retrieved 13 March 2019.
- ^ Overbye, Dennis (14 November 2019). "A Black Hole Threw a Star Out of the Milky Way Galaxy – So long, S5-HVS1, we hardly knew you". The New York Times. Retrieved 18 November 2019.
- ^ Koposov, Sergey E.; et al. (11 November 2019). "Discovery of a nearby 1700 km/s star ejected from the Milky Way by Sgr A*". Monthly Notices of the Royal Astronomical Society. arXiv:1907.11725. doi:10.1093/mnras/stz3081.
- ^ Starr, Michelle (31 July 2019). "Bizarre Star Found Hurtling Out of Our Galaxy Centre Is Fastest of Its Kind Ever Seen". ScienceAlert.com. Retrieved 18 November 2019.
- ^ Irving, Michael (13 November 2019). "Fastest star ever found is being flicked out of the Milky Way". NewAtlas.com. Retrieved 18 November 2019.
- ^ Plait, Phil (13 November 2019). "Our Local Supermassive Black Hole Shot A Star Right Out Of THe Galaxy". Bad Astronomy. Retrieved 19 November 2019.
- ^ Tauris, Thomas M. (2015). "Maximum speed of hypervelocity stars ejected from binaries". Letters. Monthly Notices of the Royal Astronomical Society. 448 (1): L6–L10. arXiv:1412.0657. Bibcode:2015MNRAS.448L...6T. doi:10.1093/mnrasl/slu189.
- ^ Maggie McKee (4 October 2008). "Milky Way's fastest stars may be immigrants". New Scientist.
- ^ Watzke, Megan (28 November 2007). "Chandra discovers cosmic cannonball". Newswise.
- ^ Shen, Ken J.; et al. (2018). "Three Hypervelocity White Dwarfs in Gaia DR2: Evidence for Dynamically Driven Double-degenerate Double-detonation Type Ia Supernovae". The Astrophysical Journal. 865 (1): 15–28. arXiv:1804.11163. Bibcode:2018ApJ...865...15S. doi:10.3847/1538-4357/aad55b. S2CID 53416740.
- ^ Zheng Zheng (7 May 2014). "Nearest Bright 'Hypervelocity Star' Found". News Center. University of Utah. Archived from the original on 1 November 2014. Retrieved 27 June 2014.
- ^ a b c d López-Santiago, J.; Montes, D.; Crespo-Chacón, I.; Fernández-Figueroa, M. J. (June 2006). "The Nearest Young Moving Groups". The Astrophysical Journal. 643 (2): 1160–1165. arXiv:astro-ph/0601573. Bibcode:2006ApJ...643.1160L. doi:10.1086/503183. S2CID 119520529.
- ^ Montes, D.; et al. (November 2001). "Late-type members of young stellar kinematic groups – I. Single stars". Monthly Notices of the Royal Astronomical Society. 328 (1): 45–63. arXiv:astro-ph/0106537. Bibcode:2001MNRAS.328...45M. doi:10.1046/j.1365-8711.2001.04781.x. S2CID 55727428.
- ^ Johnston, Kathryn V. (1996). "Fossil Signatures of Ancient Accretion Events in the Halo". The Astrophysical Journal. 465: 278. arXiv:astro-ph/9602060. Bibcode:1996ApJ...465..278J. doi:10.1086/177418. S2CID 16091481.
- ^ a b Israelian, Garik (1997). "Obituary: Victor Amazaspovich Ambartsumian, 1912 [i.e. 1908] –1996". Bulletin of the American Astronomical Society. 29 (4): 1466–1467. Bibcode:1997BAAS...29.1466I.
- ^ a b Herbst, W. (1976). "R associations. I – UBV photometry and MK spectroscopy of stars in southern reflection nebulae". Astronomical Journal. 80: 212–226. Bibcode:1975AJ.....80..212H. doi:10.1086/111734.
- ^ a b c Herbst, W.; Racine, R. (1976). "R associations. V. MON R2". Astronomical Journal. 81: 840. Bibcode:1976AJ.....81..840H. doi:10.1086/111963.
- ^ a b "OB Associations" (PDF). GAIA: Composition, Formation and Evolution of the Galaxy. 2000-04-06. Retrieved 2013-11-14.
- ^ de Zeeuw, P. T.; Hoogerwerf, R.; de Bruijne, J. H. J.; Brown, A. G. A.; et al. (1999). "A HIPPARCOS Census of the Nearby OB Associations". The Astronomical Journal. 117 (1): 354–399. arXiv:astro-ph/9809227. Bibcode:1999AJ....117..354D. doi:10.1086/300682. S2CID 16098861.
- ^ Maíz-Apellániz, Jesús (2001). "The Origin of the Local Bubble". The Astrophysical Journal. 560 (1): L83–L86. arXiv:astro-ph/0108472. Bibcode:2001ApJ...560L..83M. doi:10.1086/324016. S2CID 119338135.
- ^ Frink, S.; Roeser, S.; Neuhaeuser, R.; Sterzik, M. K. (1999). "New proper motions of pre-main-sequence stars in Taurus-Auriga". Astronomy and Astrophysics. 325: 613–622. arXiv:astro-ph/9704281. Bibcode:1997A&A...325..613F. Archived from the original on 2010-08-07. Retrieved 2009-10-12.
- ^ Herbst, W. (1975). "R-associations III. Local optical spiral structure". Astronomical Journal. 80: 503. Bibcode:1975AJ.....80..503H. doi:10.1086/111771.
- ^ Eggen, O.J. (1965). "Moving groups of stars". In Blaauw, Adriaan & Schmidt, Maarten (eds.). Observational Aspects of Galactic Structure: Lecture notes reported by participants. Chicago: University of Chicago Press. p. 111. Bibcode:1965gast.book..111E.
- ^ "BANYAN Σ". www.exoplanetes.umontreal.ca. Retrieved 2019-11-15.
- ^ a b Gagné, Jonathan; Mamajek, Eric E.; Malo, Lison; Riedel, Adric; Rodriguez, David; Lafrenière, David; et al. (2018-03-21). "The BANYAN Σ multivariate Bayesian algorithm to identify members of young associations with 150 pc". The Astrophysical Journal. BANYAN XI. 856 (1): 23. arXiv:1801.09051. Bibcode:2018ApJ...856...23G. doi:10.3847/1538-4357/aaae09. ISSN 0004-637X. S2CID 119185386.
- ^ See Gagné, Jonathan; Mamajek, Eric E.; Malo, Lison; Riedel, Adric; Rodriguez, David; Lafrenière, David; Faherty, Jacqueline K.; Roy-Loubier, Olivier; Pueyo, Laurent; Robin, Annie C.; Doyon, René (2018). "Figures 4 & 5 of Gagné et al. 2018a". The Astrophysical Journal. 856 (1): 23. arXiv:1801.09051. Bibcode:2018ApJ...856...23G. doi:10.3847/1538-4357/aaae09. S2CID 119185386.[58]
- ^ Gagné, Jonathan; Faherty, Jacqueline K.; Mamajek, Eric E. (2018-10-01). "Volans-Carina: A new 90 Myr old stellar association at 85 pc". The Astrophysical Journal. 865 (2): 136. arXiv:1808.04420. Bibcode:2018ApJ...865..136G. doi:10.3847/1538-4357/aadaed. ISSN 0004-637X. S2CID 119402144.
- ^ Zuckerman, B. (2018-12-31). "The nearby, young, Argus association: Membership, age, and dusty debris disks". The Astrophysical Journal. 870 (1): 27. arXiv:1811.01508. doi:10.3847/1538-4357/aaee66. ISSN 1538-4357. S2CID 119452542.
- ^ Torres, C.A.O.; Quast, G.R.; Melo, C.H.F.; Sterzik, M.F. (2008-08-25). Young, nearby, loose associations. arXiv:0808.3362. Bibcode:2008hsf2.book..757T in Reipurth, Bo, ed. (2008). Handbook of Star Forming Regions: Volume II, The Southern Sky. Monograph Publications (online). Volume 5. Astronomical Society of the Pacific. ISBN 978-1-58381-678-3, printed: ISBN 978-1-58381-671-4
- ^ Allers, K.N.; Liu, Michael C. (2013-07-09). "A near-infrared spectroscopic study of young field ultra-cool dwarfs". The Astrophysical Journal. 772 (2): 79. arXiv:1305.4418. Bibcode:2013ApJ...772...79A. doi:10.1088/0004-637X/772/2/79. ISSN 0004-637X. S2CID 59289573.
- ^ Schilling, Govert (January 12, 2022). "Stellar streams are revealing their secrets". Sky & Telescope. Retrieved December 13, 2022.
- ^ a b c Song, Inseok; et al. (December 2003). "New Members of the TW Hydrae Association, β Pictoris Moving Group, and Tucana/Horologium Association" (PDF). The Astrophysical Journal. 599 (1): 342–350. Bibcode:2003ApJ...599..342S. doi:10.1086/379194. S2CID 51833191.
- ^ Wylie-de Boer, Elizabeth; et al. (February 2010). "Evidence of Tidal Debris from ω Cen in the Kapteyn Group". The Astronomical Journal. 139 (2): 636–645. arXiv:0910.3735. Bibcode:2010AJ....139..636W. doi:10.1088/0004-6256/139/2/636. S2CID 119217292.
- ^ McDonald, A. R. E.; Hearnshaw, J. B. (August 1983). "The Wolf 630 moving group of stars". Monthly Notices of the Royal Astronomical Society. 204 (3): 841–852. Bibcode:1983MNRAS.204..841M. doi:10.1093/mnras/204.3.841.
Further reading
[edit]- Majewski, Steven R. (2006). "Stellar Motions". University of Virginia. Archived from the original on 2012-01-25. Retrieved 2008-02-25.
- "The Space Velocity and its Components". University of Tennessee. Archived from the original on 2008-02-16. Retrieved 2008-02-25.
- Blaauw A.; Morgan W.W. (1954). "The Space Motions of AE Aurigae and mu Columbae with Respect to the Orion Nebula". The Astrophysical Journal. 119: 625. Bibcode:1954ApJ...119..625B. doi:10.1086/145866.
- Hoogerwerf, R.; de Bruijne, J.H.J.; de Zeeuw, P.T. (2000). "The Origin of Runaway Stars". The Astrophysical Journal. 544 (2): L133. arXiv:astro-ph/0007436. Bibcode:2000ApJ...544L.133H. doi:10.1086/317315. S2CID 6725343.
- Brown; Geller; Kenyon; Kurtz (2006). "A Successful Targeted Search for Hypervelocity Stars". The Astrophysical Journal. 640 (1): L35–L38. arXiv:astro-ph/0601580. Bibcode:2006ApJ...640L..35B. doi:10.1086/503279. S2CID 17220212.
- Edelmann, H.; Napiwotzki, R.; Heber, U.; Christlieb, N.; et al. (2005). "HE 0437-5439: An Unbound Hypervelocity Main-Sequence B-Type Star". The Astrophysical Journal. 634 (2): L181–L184. arXiv:astro-ph/0511321. Bibcode:2005ApJ...634L.181E. doi:10.1086/498940. S2CID 15189914.
External links
[edit]- ESO press release about runaway stars Archived 2008-05-16 at the Wayback Machine
- Entry in the Encyclopedia of Astrobiology, Astronomy, and Spaceflight
- Two Exiled Stars Are Leaving Our Galaxy Forever
- Entry in the Encyclopedia of Astrobiology, Astronomy, and Spaceflight
- https://myspaceastronomy.com/magnetar-the-most-magnetic-stars-in-the-universe-my-space/ Archived 2023-03-06 at the Wayback Machine
- Young stellar kinematic groups, David Montes, Departamento de Astrofísica, Universidad Complutense de Madrid.