Grid energy storage
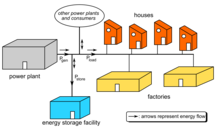
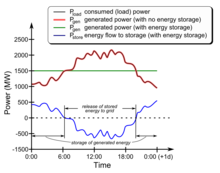
Grid energy storage (also called large-scale energy storage) is a collection of methods used for energy storage on a large scale within an electrical power grid. Electrical energy is stored during times when electricity is plentiful and inexpensive (especially from variable renewable energy sources such as wind power and solar power) or when demand is low, and later returned to the grid when demand is high, and electricity prices tend to be higher.
As of 2023[update], the largest form of grid energy storage is pumped-storage hydroelectricity, with utility-scale batteries and behind-the-meter batteries coming second and third.[1] Developments in battery storage have enabled commercially viable projects to store energy during peak production and release during peak demand, and for use when production unexpectedly falls giving time for slower responding resources to be brought online. Two forms of storage are suited for seasonal storage: green hydrogen, produced via electrolysis and thermal energy storage. As the round-trip efficiency is low, significant hydrogen needs to be stored.[2]
Energy storage is one option to making grids more flexible. An other solution is the use of more dispatchable power plants that can change their output rapidly, for instance peaking power plants to fill in supply gaps. Demand response can shift load to other times and interconnections between regions can balance out fluctuations in renewables production.[3]
Roles in the power grid
[edit]Any electrical power grid must match electricity production to consumption, both of which vary significantly over time. Energy derived from solar and wind sources varies with the weather on time scales ranging from less than a second to weeks or longer. Nuclear power is less flexible than fossil fuels, meaning it cannot easily match the variations in demand. Thus, low-carbon electricity in the absence of storage present special challenges to electric utilities.[4]
Electricity storage is one of the three key ways to replace flexibility from fossil fuels in the grid. Other options are demand-side reponse, in which consumers change when they use electricity or how much they use. For instance, consumers may have cheaper night tariffs to encourage them to use electricity at night. Industry and commercial consumers can also change their demand to meet supply. Improved network interconnection smooths the variations of renewables production and demand. When there is little wind in one location, another might have a surplus of production. Expansion of transmission lines usually takes long.[5]

Energy storage has a large set of roles in the electricity grid and can therefore provide many different services. For instance, it can move electricity from periods of low prices to high prices (arbitrage), it can help make the grid more stable, and help reduce investment into transmission infrastructure.[6] The type of service provided by storage depends on who manages the technology, whether the technology is based alongside generation of electricity, within the network, or at the side of consumption.[7]
Providing short-term flexibility is a key role for energy storage. On the generation side, it can help with the integration of variable renewable energy, storing it when there is an oversupply of wind and solar and electricity prices are low. More generally, it can exploit the changes in prices of electricity over time in the wholesale market, charging when electricity is cheap and selling when it is expensive. It can further help with grid congestion (where there is insufficient capacity on transmission lines). Consumers can use storage to use more of their self-produced electricity (for instance from rooftop solar power).[7][8]
Storage can also be used to provide essential grid services. On the generation side, storage can smooth out the variations in production, for instance for solar and wind. It can assist in a black start after a power outage. On the network side, these include frequency regulation (continuously) and frequency response (after unexpected changes in supply or demand). On the consumption side, storage can help to improve the quality of the delivered electricity in less stable grids.[7][9]
Investment in storage may make some investments in the transmission and distribution network unnecessary, or may allow them to be scaled down. Additionally, storage can ensure there is sufficient capacity to meet peak demand within the electricity grid. Finally, in off-grid home systems or mini-grids, electricity storage can help provide energy access in areas that were previously not connected to the electricity grid.[7]
Forms
[edit]Batteries
[edit]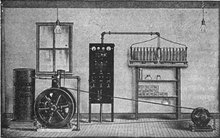

Lithium-ion batteries
[edit]Lithium-ion batteries are the most commonly used batteries for grid applications, as of 2024[update], following the application of batteries in electric vehicles (EVs). In comparison with EVs, grid batteries require less energy density, meaning that more emphasis can be put on costs, the ability to charge and discharge often and lifespan. This has led to a shift towards lithium iron phosphate batteries (LFP batteries), which are cheaper and last longer than traditional lithium-ion batteries.[13]
Costs of batteries are declining rapidly; from 2010 to 2023 costs fell by 90%.[14] As of 2024[update], utility-scale systems account for two thirds of added capacity, and home applications (behind-the-meter) for one third.[15] Lithium-ion batteries are highly suited to short-duration storage (<8h), but unlikely to become the cheapest form of electricity storage for longer-duration storage.[13]
Electric vehicles
[edit]
The electric vehicle fleet has a large overall battery capacity, which can potentially be used for grid energy storage. This could be in the form of vehicle-to-grid (V2G), where cars store energy when they are not in use, or by repurposing batteries from cars at the end of the vehicle's life. Car batteries typically range between 33 and 100 kWh;[17] for comparison, a typical upper-middle-class household in Spain might use some 18 kWh in a day.[18]
As of 2024[update], there have been more than 100 V2G pilot projects globally.[19] The effect of V2G charging on battery life can be positive or negative. Increased cycling of batteries can lead to faster degradation, but due to better management of the state of charge and gentler charging and discharing, V2G might instead increase the lifetime of batteries.[19][20] Second-hand batteries may be useable for stationary grid storage for roughly 6 years, when their capacity drops from roughly 80% to 60% of the initial capacity. LFP batteries are particularly suitable for reusing, as they degrade less than other lithium-ion batteries and recycling is less attractive as their materials are not as valuable.[19]
Other battery types
[edit]In redox flow batteries, energy is stored in liquids, which are placed in two separate tanks. When charging or discharging, the liquids are pumped into a cell with the electrodes. The amount of energy stored (as set by the size of the tanks) can be adjusted separately from the power output (as set by the speed of the pumps).[21] Flow batteries have the advantages of low capital cost for charge-discharge duration over 4 h, and of long durability (many years). Flow batteries are inferior to lithium-ion batteries in terms of energy efficiency, averaging efficiencies between 60% and 75%. Vanadium redox batteries is most commercially advanced type of flow battery, with roughly 40 companies making them as of 2022[update].[22]
Sodium-ion batteries are possible alternative to lithium-ion batteries, as they rely on cheaper materials and less on critical materials. It has a lower energy density, and possibly a shorter lifespan. If produced at the same scale as lithium-ion batteries, they may become 20% to 30% cheaper.[21] Iron-air batteries may be suitable for even longer duration storage than flow batteries (weeks), but the technology is not yet mature.[23]
Technology | Less than 4h | 4h to 8h | Days | Weeks | Seasons |
---|---|---|---|---|---|
Lithium-ion | Yes | Yes | No | No | No |
Sodium-ion | Yes | Yes | No | No | No |
Vanadium flow | Maybe | Yes | Yes | No | No |
Iron-air | No | No | Maybe | Yes | No |
Electrical
[edit]Storage in supercapacitors works well for applications where a lot of power is needed for short amount of time. In the power grid, they are therefore mostly used in short-term frequency regulation.[24]
Hydrogen and chemical storage
[edit]Various power-to-gas technologies exist that can convert excess electricity into an easier to store chemical. The lowest cost and most efficient one is hydrogen. However, it is easier to use synthetic methane with existing infrastructure and appliances, as it is very similar to natural gas.[25]
As of 2024[update], there have been a number of demonstration plants where hydrogen is burned in gas turbines, either co-firing with natural gas, or on its own. Similarly, a number of coal plants have demonstrated it is possible to co-fire ammonia when burning coal. In 2022, there was also a small pilot to burn pure ammonia in a gas turbine.[26] A portion of existing gas turbines are capable of co-firing hydrogen, which means there is, as a lower estimate, 80 GW of capacity ready to burn hydrogen.[27]
Hydrogen
[edit]Hydrogen can be used as a long-term storage medium.[28] Green hydrogen is produced from the electrolysis of water, and converted back into electricity in an internal combustion engine, or a fuel cell, with a round-trip efficiency of roughly 41%.[29] Together with thermal storage, it is expected to be best suited to seasonal energy storage.[30]
The price ratio between purchase and sale of electricity must be at least proportional to the efficiency in order for the system to be economic. Whether hydrogen can use natural gas infrastructure depends on the network construction materials, standards in joints, and storage pressure.[31]
Hydrogen can be stored aboveground in tanks or underground in larger quantities. Underground storage is easiest in salt caverns, but only a certain number of places have suitable geology.[32] Storage in porous rocks, for instance in empty gas fields and some aquifers, can store hydrogen at a larger scale, but this type of storage may have some drawbacks. For instance, some of the hydrogen may leak, or react into H2S or methane.[33]
Ammonia
[edit]Hydrogen can be converted into ammonia in a reaction with nitrogen in the Haber-Bosch process. Ammonia, a gas at room temperature, is more expensive to produce than hydrogen. However, it can be stored more cheaply than hydrogen. Tank storage is usually done at between one and ten times atmospheric pressure and at a temperature of −30 °C (−22 °F), in liquid form.[34] Ammonia can be burned cleanly: water and nitrogen are released, but no CO2 and little or no nitrogen oxides.[citation needed] Ammonia has multiple uses besides being an energy carrier: it is the basis for the production of many chemicals; the most common use is for fertilizer.[35] Just like natural gas, the stored ammonia can be used as a thermal fuel for transportation and electricity generation or used in a fuel cell.[36]
Methane
[edit]It is possible to further convert hydrogen into methane via the Sabatier reaction, a chemical reaction which combines CO2 and H2. While the reaction that converts CO from gasified coal into CH4 is mature, the process to form methane out of CO2 is less so. Efficiencies of around 80% one-way can be achieved, that is, some 20% of the energy in hydrogen is lost in the reaction.[37]
Mechanical
[edit]Flywheel
[edit]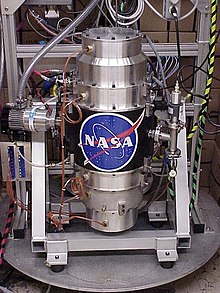
Flywheels store energy in the form of mechanical energy. They are suited to supplying high levels of electricity over minutes and can also be charged rapidly. They have a long lifetime and can be used in settings with widely varying temperatures. The technology is mature, but more expensive than batteries and supercapacitors and not used frequently.[38]
Pumped hydro
[edit]In 2023, world pumped hydroelectric storage (PHS) was the largest storage technology, with a capacity of 181 GW, compared to some 55 GW of storage in utility-scale batteries and 33 GW of behind-the-meter batteries.[39] PHS is well suited to evening out daily variations, pumping water to a high storage reservoir during off-peak hours, and using this water during peak times for hydroelectric generation.[40] The efficiency of PHS ranges between 75% and 85%, and the response time is fast, between seconds and minutes.[41]
PHS systems can only be built in limited locations. Pumped storage systems may also be possible by using deep salt caverns or building a hollow deposit at the seabed, and using the sea itself as the higher reservoir.[40] PHS construction can be costly, takes relatively long and can be disruptive for the environment and people living nearby.[40] The efficiency of pumped hydro can be increased by placing floating solar panels on top, which prevent evaporation. This also improves the efficiency of the solar panels, as they are constantly cooled.[42]
Hydroelectric dams
[edit]
Hydroelectric dams with large reservoirs can also be operated to provide peak generation at times of peak demand. Water is stored in the reservoir during periods of low demand and released through the plant when demand is higher. While technically no electricity is stored, the net effect is the similar as pumped storage. The amount of storage available in hydroelectric dams is much larger than in pumped storage. Upgrades may be needed so that these dams can respond to variable demand. For instance, additional investment may be needed in transmission lines, or additional turbines may need to be installed to increase the peak output from the dam.[43]
Dams usually have multiple purposes. As well as energy generation, they often play a role in flood defense and protection of ecosystems, recreation, and they supply water for irrigation. This means it is not always possible to change their operation much, but even with low flexibility, they may still play an important role in responding to changes in wind and solar production.[44]
Gravity
[edit]Alternatives include storing energy by moving large solid masses upward against gravity. This can be achieved inside old mine shafts[45] or in specially constructed towers where heavy weights are winched up to store energy and allowed a controlled descent to release it.[46][47]
Compressed air
[edit]Compressed air energy storage (CAES) stores electricity by compressing air. The compressed air is typically stored in large underground caverns. The expanding air can be used to drive turbines, converting the energy back into electricity. As air cools when expanding, some heat needs to be added in this stage to prevent freezing. This can be provided by heat stored from a low-carbon source, or in the case of advanced CAES, from reusing the heat that is released when air is compressed. As of 2023[update], there are three advanced CAES project in operation in China.[48] Typical efficiencies of advanced CAES are between 60% and 80%.[49]
Liquid air or CO2
[edit]Another electricity storage method is to compress and cool air, turning it into liquid air, which can be stored, and expanded when needed, turning a turbine, generating electricity. This is called liquid air energy storage (LAES).[50] The air would be cooled to temperatures of −196 °C (−320.8 °F) to become liquid. Like with compressed air, heat is needed for the expansion step. In the case of LAES, low-grade industrial heat can be used for this.[38] Energy efficiency for LEAS lies between 50% and 70%. As of 2023[update], LAES is moving from pre-commercial to commercial.[51]
Italian firm Energy Dome uses supercritical (liquified by compression) CO
2 drawn from an atmospheric gasholder. Energy is accessed by evaporating and expanding the CO
2 into a turbine. The gas is returned to the atmospheric gasholder, until the next charging cycle. The system can be run in a closed loop, avoiding emissions. In July, 2024, the US DOE Office of Clean Energy Demonstrations awarded $7 million to an Energy Dome test project hosted by US gas and electricity supplier Alliant Energy.[52]
Thermal
[edit]Electricity can be directly stored thermally with a Carnot battery. A Carnot battery is a type of energy storage systems that stores electricity in heat storage and converts the stored heat back to electricity via thermodynamics cycles (for instance, a turbine). While less efficient than pumped hydro or battery storage, this type of system is expected to be cheap and can provide long duration storage.[53][54] A pumped-heat electricity storage system is a Carnot battery that uses a reversible heat pump to pump convert the electricity into heat.[55] It usually stores the energy in both a hot and cold reservoir. To achieve decent efficiencies (>50%), the temperature ratio between the two must reach a factor of 5.[56]
Thermal energy storage is also used in combination with concentrated solar power (CSP). In CSP, Solar energy is first converted into heat, and then either directly converted into electricity or first stored. The energy is released when there is little or no sunshine.[57] This means that CSP can be used as a dispatchable (flexible) form of generation. The energy in a CSP system can for instance be stored in molten salts or in a solid medium such as sand.[58]
Finally, building heating and cooling systems can be controlled to store thermal energy in either the building's mass or dedicated thermal storage tanks. This thermal storage can provide load-shifting or even more complex ancillary services by increasing power consumption (charging the storage) during off-peak times and lowering power consumption (discharging the storage) during higher-priced peak times.[59]
Economics
[edit]The levelized cost of storing electricity depends highly on storage type and purpose; as subsecond-scale frequency regulation, minute/hour-scale peaker plants, or day/week-scale season storage.[60][61][62] Using battery storage is said to have a levelized cost of $120[63] to $170[64] per MWh. This compares with open cycle gas turbines which, as of 2020, have a cost of around $151–198 per MWh.[65]
Electricity storage is not 100% efficient, so more electricity needs to be bought than can be sold. This implies that if there is only a small variation in price, it may not be economical to charge and discharge. For instance, if the storage application is 75% efficient, the price at which the electricity is sold needs to be at least 1.33 higher than the price for which it was bought.[66]
However, the marginal cost of electricity varies because of the varying operational and fuel costs of different classes of generators.[67] At one extreme, base load power plants such as coal-fired power plants and nuclear power plants are low marginal cost generators, as they have high capital and maintenance costs but low fuel costs. At the other extreme, peaking power plants such as gas turbine natural gas plants burn expensive fuel but are cheaper to build, operate and maintain. To minimize the total operational cost of generating power, base load generators are dispatched most of the time, while peak power generators are dispatched only when necessary, generally when energy demand peaks. This is called "economic dispatch".
Demand for electricity from the world's various grids varies over the course of the day and from season to season. For the most part, variation in electric demand is met by varying the amount of electrical energy supplied from primary sources. Increasingly, however, operators are storing lower-cost energy produced at night, then releasing it to the grid during the peak periods of the day when it is more valuable.[68]
It may be more economical to find an alternative market for unused electricity, rather than try and store it. High Voltage Direct Current allows for transmission of electricity, losing only 3% per 1000 km.
Load leveling
[edit]The demand for electricity from consumers and industry is constantly changing, broadly within the following categories:
- Seasonal (during dark winters more electric lighting and heating is required, while in other climates hot weather boosts the requirement for air conditioning)
- Weekly (most industry closes at the weekend, lowering demand)
- Daily (such as the morning peak as offices open and air conditioners get switched on)
- Hourly (one method for estimating television viewing figures in the United Kingdom is to measure the power spikes during advertisement breaks or after programmes when viewers go to switch a kettle on)[69]
- Transient (fluctuations due to individuals' actions, differences in power transmission efficiency and other small factors that need to be accounted for)
There are currently three main methods for dealing with changing demand:
- Electrical devices generally having a working voltage range that they require, commonly 110–120 V or 220–240 V. Minor variations in load are automatically smoothed by slight variations in the voltage available across the system.
- Power plants can be run below their normal output, with the facility to increase the amount they generate almost instantaneously. This is termed "spinning reserve".
- Additional generation can be brought online. Typically, these would be hydroelectric or gas turbines, which can be started in a matter of minutes.
The problem with standby gas turbines is higher costs; expensive generating equipment is unused much of the time. Spinning reserve also comes at a cost; plants running below maximum output are usually less efficient. Grid energy storage is used to shift generation from times of peak load to off-peak hours. Power plants are able to run at their peak efficiency during nights and weekends.
Supply-demand leveling strategies may be intended to reduce the cost of supplying peak power or to compensate for the intermittent generation of wind and solar power.
See also
[edit]- Battery electric vehicles
- Cost of electricity by source
- Distributed generation
- Energy demand management
- Energy storage as a service (ESaaS)
- Gravity battery
- Hydrogen economy
- List of energy storage projects
- Power-to-X
- Rechargeable battery
- U.S. Department of Energy International Energy Storage Database, a list of grid energy storage projects
- Virtual power plant
References
[edit]- ^ Cozzi, Petropoulos & Wanner 2024, p. 68.
- ^ IPCC AR6 WG3 Ch6 2022, pp. 653, 656.
- ^ IPCC AR6 WG3 Ch6 2022, p. 651.
- ^ Schmidt & Staffell 2023, p. 8.
- ^ Schmidt & Staffell 2023, pp. 10–11.
- ^ Armstrong & Chiang 2022, pp. 6–7.
- ^ a b c d Cozzi, Petropoulos & Wanner 2024, p. 36.
- ^ Schmidt & Staffell 2023, pp. 74–76.
- ^ Schmidt & Staffell 2023, pp. 74–75.
- ^ Hawkins, Nehemiah (1917). Hawkins Electrical Guide ...: Questions, Answers & Illustrations; a Progressive Course of Study for Engineers, Electricians, Students and Those Desiring to Acquire a Working Knowledge of Electricity and Its Applications; a Practical Treatise. T. Audel & Company. pp. 989–.
- ^ Ziegler, Micah S.; Trancik, Jessika E. (2021). "Re-examining rates of lithium-ion battery technology improvement and cost decline". Energy & Environmental Science. 14 (4): 1635–1651. arXiv:2007.13920. doi:10.1039/D0EE02681F. ISSN 1754-5692. S2CID 220830992.
- ^ "The price of batteries has declined by 97% in the last three decades". Our World in Data. Retrieved 26 April 2022.
- ^ a b Cozzi, Petropoulos & Wanner 2024, p. 45.
- ^ Cozzi, Petropoulos & Wanner 2024, p. 18.
- ^ Cozzi, Petropoulos & Wanner 2024, p. 20.
- ^ "Nissan and Ecobat to give used EV batteries a second life beyond the car". Official Great Britain Newsroom. 30 April 2024. Retrieved 21 November 2024.
- ^ Xu, Chengjian; Behrens, Paul; Gasper, Paul; Smith, Kandler; Hu, Mingming; Tukker, Arnold; Steubing, Bernhard (17 January 2023). "Electric vehicle batteries alone could satisfy short-term grid storage demand by as early as 2030". Nature Communications. 14 (1). doi:10.1038/s41467-022-35393-0. ISSN 2041-1723. PMC 9845221.
- ^ García-Vázquez, Carlos Andrés; Espinoza-Ortega, Hernán; Llorens-Iborra, Francisco; Fernández-Ramírez, Luis M. (1 November 2022). "Feasibility analysis of a hybrid renewable energy system with vehicle-to-home operations for a house in off-grid and grid-connected applications". Sustainable Cities and Society. 86: 104124. doi:10.1016/j.scs.2022.104124. ISSN 2210-6707.
- ^ a b c Aguilar Lopez, Fernando; Lauinger, Dirk; Vuille, François; Müller, Daniel B. (16 May 2024). "On the potential of vehicle-to-grid and second-life batteries to provide energy and material security". Nature Communications. 15 (1). doi:10.1038/s41467-024-48554-0. ISSN 2041-1723. PMC 11099178.
- ^ Bhoir, Shubham; Caliandro, Priscilla; Brivio, Claudio (1 December 2021). "Impact of V2G service provision on battery life". Journal of Energy Storage. 44: 103178. doi:10.1016/j.est.2021.103178. ISSN 2352-152X.
- ^ a b Cozzi, Petropoulos & Wanner 2024, p. 46.
- ^ Tolmachev, Yuriy V. (1 March 2023). "Review—Flow Batteries from 1879 to 2022 and Beyond". Journal of the Electrochemical Society. 170 (3): 030505. Bibcode:2023JElS..170c0505T. doi:10.1149/1945-7111/acb8de. ISSN 0013-4651.
- ^ a b Cozzi, Petropoulos & Wanner 2024, p. 47.
- ^ Schmidt & Staffell 2023, pp. 54–55.
- ^ Letcher 2022, p. 606.
- ^ Remme & Bermudez Menendez 2024, pp. 54–55.
- ^ Remme & Bermudez Menendez 2024, p. 57.
- ^ Smith 2023, p. 5.
- ^ Smith 2023, p. 14.
- ^ IPCC AR6 WG3 Ch6 2022, p. 653.
- ^ "Conversion of the UK gas system to transport hydrogen". Archived from the original on 16 May 2016.
- ^ Armstrong & Chiang 2022, p. 150.
- ^ Miocic, Johannes; Heinemann, Niklas; Edlmann, Katriona; Scafidi, Jonathan; Molaei, Fatemeh; Alcalde, Juan (30 August 2023). "Underground hydrogen storage: a review". Geological Society, London, Special Publications. 528 (1): 73–86. doi:10.1144/SP528-2022-88. hdl:10261/352537. ISSN 0305-8719.
- ^ Smith 2023, p. 18.
- ^ Service, Robert F. (12 July 2018). "Ammonia—a renewable fuel made from sun, air, and water—could power the globe without carbon". Science | AAAS. Retrieved 15 April 2021.
- ^ Lan, Rong; Tao, Shanwen (5 May 2018). "Ammonia as a Suitable Fuel for Fuel Cells". Frontiers in Energy Research. 2. doi:10.3389/fenrg.2014.00035.
- ^ Letcher 2022, p. 602.
- ^ a b IPCC AR6 WG3 Ch6 2022, p. 655.
- ^ "Global installed energy storage capacity by scenario, 2023 and 2030 – Charts – Data & Statistics". International Energy Agency. Retrieved 25 August 2024.
- ^ a b c IPCC AR6 WG3 Ch6 2022, p. 654.
- ^ Javed, Muhammad Shahzad; Ma, Tao; Jurasz, Jakub; Amin, Muhammad Yasir (1 April 2020). "Solar and wind power generation systems with pumped hydro storage: Review and future perspectives". Renewable Energy. 148: 176–192. doi:10.1016/j.renene.2019.11.157. ISSN 0960-1481.
- ^ IRENA 2020, p. 7.
- ^ Armstrong & Chiang 2022, pp. 69–70.
- ^ Armstrong & Chiang 2022, pp. 69.
- ^ "How UK's disused mine shafts could be used to store renewable energy". The Guardian. 21 October 2019.
- ^ Gourley, Perry (31 August 2020). "Edinburgh firm behind incredible gravity energy storage project hails milestone". www.edinburghnews.scotsman.com. Retrieved 1 September 2020.
- ^ Akshat Rathi (18 August 2018). "Stacking concrete blocks is a surprisingly efficient way to store energy". Quartz.
- ^ Smith 2023, p. 19.
- ^ Zhang, Xinjing; Gao, Ziyu; Zhou, Bingqian; Guo, Huan; Xu, Yujie; Ding, Yulong; Chen, Haisheng (2024). "Advanced Compressed Air Energy Storage Systems: Fundamentals and Applications". Engineering. 34: 246–269. doi:10.1016/j.eng.2023.12.008. ISSN 2095-8099.
- ^ Smith 2023, p. 20.
- ^ Liang, Ting; Zhang, Tongtong; Lin, Xipeng; Alessio, Tafone; Legrand, Mathieu; He, Xiufen; Kildahl, Harriet; Lu, Chang; Chen, Haisheng; Romagnoli, Alessandro; Wang, Li; He, Qing; Li, Yongliang; Yang, Lizhong; Ding, Yulong (2023). "Liquid air energy storage technology: a comprehensive review of research, development and deployment". Progress in Energy. 5 (1): 012002. doi:10.1088/2516-1083/aca26a. ISSN 2516-1083.
- ^ Casey, Tina (26 August 2024). "Giant Bubble Of CO2 For Long Duration Energy Storage". CleanTechnica. Retrieved 28 August 2024.
- ^ Dumont, Olivier; Frate, Guido Francesco; Pillai, Aditya; Lecompte, Steven; De paepe, Michel; Lemort, Vincent (2020). "Carnot battery technology: A state-of-the-art review". Journal of Energy Storage. 32: 101756. Bibcode:2020JEnSt..3201756D. doi:10.1016/j.est.2020.101756. hdl:2268/251473. ISSN 2352-152X. S2CID 225019981.
- ^ Vandersickel & Gutierrez 2023, p. 107.
- ^ Benato, Alberto; Stoppato, Anna (1 June 2018). "Pumped Thermal Electricity Storage: A technology overview". Thermal Science and Engineering Progress. 6: 301–315. doi:10.1016/j.tsep.2018.01.017. ISSN 2451-9049.
- ^ Albertus, Paul; Manser, Joseph S.; Litzelman, Scott (2020). "Long-Duration Electricity Storage Applications, Economics, and Technologies". Joule. 4 (1): 21–32. doi:10.1016/j.joule.2019.11.009. ISSN 2542-4351.
- ^ Pelay, Ugo; Luo, Lingai; Fan, Yilin; Stitou, Driss; Rood, Mark (1 November 2017). "Thermal energy storage systems for concentrated solar power plants". Renewable and Sustainable Energy Reviews. 79: 82–100. doi:10.1016/j.rser.2017.03.139. ISSN 1364-0321.
- ^ "Thermal Storage System Concentrating Solar-Thermal Power Basics". Energy.gov. Retrieved 17 November 2024.
- ^ Lee, Zachary E.; Sun, Qingxuan; Ma, Zhao; Wang, Jiangfeng; MacDonald, Jason S.; Zhang, K. Max (February 2020). "Providing Grid Services With Heat Pumps: A Review". Journal of Engineering for Sustainable Buildings and Cities. 1 (1). doi:10.1115/1.4045819. S2CID 213898377.
- ^ "Some energy storage already cost competitive, new valuation study shows". Utility Dive. 24 November 2015. Archived from the original on 18 October 2016. Retrieved 15 October 2016.
- ^ "Lazard's Levelized Cost of Storage Analysis" (PDF). Archived (PDF) from the original on 2 February 2017. Retrieved 2 February 2017.
- ^ Lai, Chun Sing; McCulloch, Malcolm D. (March 2017). "Levelized cost of electricity for solar photovoltaic and electrical energy storage". Applied Energy. 190: 191–203. Bibcode:2017ApEn..190..191L. doi:10.1016/j.apenergy.2016.12.153. S2CID 113623853.
- ^ Chip Register (13 January 2015). "The Battery Revolution: A Technology Disruption, Economics and Grid Level Application Discussion with Eos Energy Storage". Forbes. Archived from the original on 11 November 2016.
- ^ "Eos Energy Storage – Technology and Products". eosenergystorage.com. Archived from the original on 6 February 2014.
- ^ "Levelized Cost of Energy and of Storage". Archived from the original on 20 February 2021. Retrieved 5 January 2021.
- ^ Schmidt & Staffell 2023, p. 191.
- ^ Lai, Chun Sing; Jia, Youwei; Xu, Zhao; Lai, Loi Lei; Li, Xuecong; Cao, Jun; McCulloch, Malcolm D. (December 2017). "Levelized cost of electricity for photovoltaic/biogas power plant hybrid system with electrical energy storage degradation costs". Energy Conversion and Management. 153: 34–47. Bibcode:2017ECM...153...34L. doi:10.1016/j.enconman.2017.09.076.
- ^ Energy Information Administration / Annual Energy Review 2006 Archived 25 June 2008 at the Wayback Machine, Table 8.2a
- ^ "BBC News – Christmas Television – The great TV ratings war". BBC. Archived from the original on 12 January 2009.
Cited sources
[edit]- Armstrong, Robert; Chiang, Yet-Ming (2022). The Future of Energy Storage: An Interdisciplinary MIT Study (PDF). Massachusetts Institute of Technology. ISBN 978-0-578-29263-2.
- Cozzi, Laura; Petropoulos, Apostolos; Wanner, Brent (April 2024). Batteries and Secure Energy Transitions (PDF). International Energy Agency.
- Remme, Uwe; Bermudez Menendez, Jose Miguel; et al. (October 2024). Global Hydrogen Review 2024 (PDF). International Energy Agency.
- Clarke, L.; Wei, Y.-M.; De La Vega Navarro, A.; Garg, A.; et al. "Chapter 6: Energy Systems" (PDF). Climate Change 2022: Mitigation of Climate Change. Contribution of Working Group III to the Sixth Assessment Report of the Intergovernmental Panel on Climate Change (Report). doi:10.1017/9781009157926.008.
- IRENA (2020). Innovation landscape brief: Innovative operation of pumped hydropower storage. Abu Dhabi: International Renewable Energy Agency. ISBN 978-92-9260-180-5.
- Letcher, Trevor M., ed. (2022). Storing energy: with special reference to renewable energy sources (Second ed.). Amsterdam Oxford Cambridge, MA: Elsevier. ISBN 978-0-12-824510-1.
- Schmidt, Oliver; Staffell, Iain (2023). Monetizing energy storage: a toolkit to assess future cost and value. Oxford, United Kingdom: Oxford University Press. ISBN 978-0-19-288817-4.
- Smith, Chris Llewellyn (2023). Large-scale electricity storage (PDF). Royal Society. ISBN 978-1-78252-666-7.
- Vandersickel, Annelies; Gutierrez, Andrea (2023). Task 36 Carnot Batteries Final Report (Report). Technology Collaboration Programme Energy Storage, International Energy Agency. Retrieved 29 October 2024.