User:WilsonDiep/sandbox
Coral reef restoration has grown in prominence over the past several decades because of the unprecedented reef die offs around the planet. Coral stressors can include pollution, warming ocean temperatures, extreme weather events, and overfishing. With the deterioration of global reefs, fish nurseries, biodiversity, coastal development and livelihood, and natural beauty are under threat. Fortunately, researchers have taken it upon themselves to develop a new field, coral restoration, in the 1970s-1980s[1]
Coral aquaculture, also known as coral farming or coral gardening, is showing promise as a potentially effective tool for restoring coral reefs. The "gardening" process bypasses the early growth stages of corals when they are most at risk of dying. Coral seeds are grown in nurseries, then replanted on the reef. Coral is farmed by coral farmers whose interests range from reef conservation to increased income. Due to its straight forward process and substantial evidence of the technique having a significant effect on coral reef growth, coral nurseries became the most widespread and arguably the most effective method for coral restoration[2].

Coral gardens take advantage of a coral's natural ability to fragment and continuing to grow if the fragments are able to anchor themselves onto new substrates. This method was first tested by Baruch Rinkevich [3] in 1995 which found sucess at the time. By today's standards, coral farming has grown into a variety of different forms, but still have the same goals of cultivating corals. Consequently, coral farming quickly replaced previously used transplantation methods, or the act of physically moving sections or whole colonies of corals into a new area[2]. Transplantation has seen success in the past and decades of experiments have led to a high success and survival rate. However, this method still requires the removal of corals from existing reefs. With the current state of reefs, this kind of method should generally be avoided if possible. Saving healthy corals from eroding substrates or reefs that are doomed to collapse could be a major advantage of utilizing transplantation.
Coral gardens generally take on the safe forms no matter where you go. It begins with the establishment of a nursery where operators can observe and care for coral fragments. It goes without saying that nurseries should be established in areas that are going to maximize growth and minimize mortality. Floating offshore coral trees or even aquariums are possible locations where corals can grow. After a location has been determined, collection and cultivation can occur.
The major benefit for using coral farms is it lowers polyp and juvenile mortality rates. By removing predators and recruitment obstacles, corals are able to mature without much hindrance. However, it should be noted that nurseries do cannot stop climate stressors. Warming temperatures or hurricanes can still disrupt or even outright kill nursery corals.
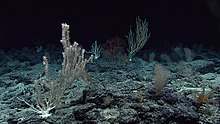
Efforts to expand the size and number of coral reefs generally involve supplying substrate to allow more corals to find a home. Substrate materials include discarded vehicle tires, scuttled ships, subway cars and formed concrete, such as reef balls. Reefs grow unaided on marine structures such as oil rigs. In large restoration projects, propagated hermatypic coral on substrate can be secured with metal pins, superglue or milliput. Needle and thread can also attach A-hermatype coral to substrate.
Biorock is a substrate produced by a patented process that runs low voltage electrical currents through seawater to cause dissolved minerals to precipitate onto steel structures. The resultant white carbonate (aragonite) is the same mineral that makes up natural coral reefs. Corals rapidly colonize and grow at accelerated rates on these coated structures. The electrical currents also accelerate formation and growth of both chemical limestone rock and the skeletons of corals and other shell-bearing organisms, such as oysters. The vicinity of the anode and cathode provides a high-pH environment which inhibits the growth of competitive filamentous and fleshy algae. The increased growth rates fully depend on the accretion activity. Under the influence of the electric field, corals display an increased growth rate, size and density.
Simply having many structures on the ocean floor is not enough to form coral reefs. Restoration projects must consider the complexity of the substrates they are creating for future reefs. Researchers conducted an experiment near Ticao Island in the Philippines in 2013[4] where several substrates in varying complexities were laid in the nearby degraded reefs. Large complexity consisted of plots that had both a man made substrates of both smooth and rough rocks with a surrounding fence, medium consisted of only the man made substrates, and small had neither the fence or substrates. After one month, researchers found that there was positive correlation between structure complexity and recruitment rates of larvae[4]. The medium complexity performed the best with larvae favoring rough rocks over smooth rocks. Following one year of their study, researchers visited the site and found that many of the sites were able to support local fisheries. They came to the conclusion that reef restoration could be done cost effectively and will yield long term benefits given they are protected and maintained.[4]
One case study with coral reef restoration was conducted on the island of Oahu in Hawaii. The University of Hawaii operates a Coral Reef Assessment and Monitoring Program to help relocate and restore coral reefs in Hawaii. A boat channel from the island of Oahu to the Hawaii Institute of Marine Biology on Coconut Island was overcrowded with coral reefs. Many areas of coral reef patches in the channel had been damaged from past dredging in the channel.

Dredging covers corals with sand. Coral larvae cannot settle on sand; they can only build on existing reefs or compatible hard surfaces, such as rock or concrete. Because of this, the University decided to relocate some of the coral. They transplanted them with the help of United States Army divers, to a site relatively close to the channel. They observed little if any damage to any of the colonies during transport and no mortality of coral reefs was observed on the transplant site. While attaching the coral to the transplant site, they found that coral placed on hard rock grew well, including on the wires that attached the corals to the site.
No environmental effects were seen from the transplantation process, recreational activities were not decreased, and no scenic areas were affected.
As an alternative to transplanting coral themselves, juvenile fish can also be encouraged to relocate to existing coral reefs by auditory simulation. In damaged sections of the Great Barrier Reef, loudspeakers playing recordings of healthy reef environments, were found to attract fish twice as often as equivalent patches where no sound was played, and also increased species biodiversity by 50%.
Another possibility for coral restoration is gene therapy: inoculating coral with genetically modified bacteria, or naturally-occurring heat-tolerant varieties of coral symbiotes, may make it possible to grow corals that are more resistant to climate change and other threats. Warming oceans are forcing corals to adapt the to a unprecedented temperatures. Those that do not have a tolerance for the elevated temperatures experience coral bleaching and eventually mortality. There is already research that looks to create genetically modified corals that can withstand a warming ocean. Madeleine J. H. van Oppen, James K. Oliver, Hollie M. Putnam, and Ruth D. Gates described four different ways that gradually increase in human intervention to genetically modify corals [5]. These methods focus on altering the genetics of the zooxanthellae within coral rather than the alternative.
The first method is to induced acclimatization of the first generation of corals[5]. The idea is that when adult and offspring corals are exposed to stressors, the zooxanthellae will gain a mutation. This method is based mostly on the chance that the zooxanthellae will acquire the specific trait that will allow it to better survive in warmer waters. The second method focuses on identifying what different kinds of zooxanthellae are within the coral and configuring how much of each zooxanthellae lives within to the coral at a given age[5]. Use of zooxanthellae from the previous method would only boost boost success rates for this method. However, this method would only be applicable to younger corals, for now, because previous experiments of manipulation zooxanthellae communities at later life stages have all failed. The third method focuses on selective breeding tactics[5]. Once selected, corals would be reared and exposed to simulated stressors in a laboratory. The last method is to genetically modify the zooxanthellae itself[5]. When preferred mutations are acquired, the genetically modified zooxanthellae will be introduced to an aposymbiotic poly and a new coral will be produced. This method is the most laborious of the fourth, but researchers believe this method should be utilized more and holds the most promise in genetic engineering for coral restoration.
Hawaiian coral reefs smothered by the spread of invasive algae were managed with a two-prong approach: divers manually removed invasive algae, with the support of super-sucker barges. Grazing pressure on invasive algae needed to be increased to prevent the regrowth of the algae.
Invasive Algae in Caribbean Reefs
[edit]Macroalgae, or better known as seaweed, has to potential to cause reef collapse because they can outcompete many coral species. Macroalgae can overgrow on corals, shade, block recruitment, release biochemicals that can hinder spawning, and potentially form bacteria harmful to corals. [6][7] Historically, algae growth was controlled by herbivorous fish and sea urchins. Parrotfish are a prime example of reef caretakers. Consequently, these two species can be considered as keystone species for reef environments because of their role in protecting reefs.
Before the 1980s, Jamaica's reefs were thriving and well cared for, however this all changed after Hurricane Allen occurred in 1980 and an unknown disease spread across the Caribbean. In the wake of these events, massive damage was caused to both the reefs and sea urchin population across Jamaican's reefs and into the Caribbean Sea. As little as 2% of the original sea urchin population survived the disease. [7] Primary macroalgae succeeded the destroyed reefs and eventually larger, more resilient macroalgae soon took its place as the dominant organism.[7][8] Parrotfish and other herbivorous fish were few in numbers because of decades of overfishing and bycatch at the time.[8] Historically, the Jamaican coast had 90% coral cover and was reduced to 5% in the 1990s.[8] Eventually, corals were able to recover in areas where sea urchin populations were increasing. Sea urchins were able to feed and multiply and clear off substrates, leaving areas for coral polyps to anchor and mature. However, sea urchin populations are still not recovering as fast as researchers predicted, despite being highly fecundate. [7] It is unknown whether or not the mysterious disease is still present and preventing sea urchin populations from rebounding. Regardless, these areas are slowly recovering with the aid of sea urchin grazing. This event supports an early restoration idea of cultivating and releasing sea urchins into reefs to prevent algal overgrowth. [9][10]
In 2014, Christopher Page, Erinn Muller, and David Vaughan from the International Center for Coral Reef Research & Restoration at Mote Marine Laboratory in Summerland Key, Florida developed a new technology called "microfragmentation," in which they use a specialized diamond band saw to cut corals into 1 cm2 fragments instead of 6 cm2 to advance the growth of brain, boulder, and star corals[11]. Corals Orbicella faveolata and Montastraea cavernosa were outplanted off the Florida's shores in several microfragment arrays. After two years, O. faveolata had grown 6.5x its original size while M. cavernosa had grown nearly twice its size[11]. Under conventional means, both corals would have required decades to reach the same size. It is suspected that if predation events had not occurred near the beginning of the experiment O. faveolata would have grown at least 10x it's original size[11]. By using this method, Mote Marine Laboratory produced 25,000 corals and planted 10,000 in the Florida Keys in only one year. Shortly after, they discovered that these microfragments fused with other microfragments from the same parent coral. Typically, corals that are not from the same parent fight and kill nearby corals in an attempt to survive and expand. This new technology is known as "fusion" and has been shown to grow coral heads in just two years instead of the typical 25–75 years. After fusion occurs, the reef will act as a single organism rather than several independent reefs. Currently, there has been no published research into this method[11].
***
**
- ^ "Coral Restoration – Shark Research & Conservation Program (SRC) | University of Miami". Retrieved 2020-05-03.
- ^ a b Lirman, Diego; Schopmeyer, Stephanie (2016-10-20). "Ecological solutions to reef degradation: optimizing coral reef restoration in the Caribbean and Western Atlantic". PeerJ. 4: e2597. doi:10.7717/peerj.2597. ISSN 2167-8359.
{{cite journal}}
: CS1 maint: unflagged free DOI (link) - ^ Rinkevich, Baruch (1995). "Restoration Strategies for Coral Reefs Damaged by Recreational Activities: The Use of Sexual and Asexual Recruits". Restoration Ecology. 3 (4): 241–251. doi:10.1111/j.1526-100X.1995.tb00091.x. ISSN 1526-100X.
- ^ a b c Yanovski, Roy; Abelson, Avigdor (2019-07-01). "Structural complexity enhancement as a potential coral-reef restoration tool". Ecological Engineering. 132: 87–93. doi:10.1016/j.ecoleng.2019.04.007. ISSN 0925-8574.
- ^ a b c d e Oppen, Madeleine J. H. van; Oliver, James K.; Putnam, Hollie M.; Gates, Ruth D. (2015-02-24). "Building coral reef resilience through assisted evolution". Proceedings of the National Academy of Sciences. 112 (8): 2307–2313. doi:10.1073/pnas.1422301112. ISSN 0027-8424. PMID 25646461.
- ^ Vieira, Christophe; Payri, Claude; Clerck, Olivier (2016-09-08). "A fresh look at macroalgal-coral interactions: are macroalgae a threat to corals?". Perspectives in Phycology. 3. doi:10.1127/pip/2016/0068.
- ^ a b c d Knowlton, N. (2001-04-24). "Sea urchin recovery from mass mortality: New hope for Caribbean coral reefs?". Proceedings of the National Academy of Sciences. 98 (9): 4822–4824. doi:10.1073/pnas.091107198. ISSN 0027-8424.
- ^ a b c Edmunds, P. J.; Carpenter, R. C. (2001-03-27). "Recovery of Diadema antillarum reduces macroalgal cover and increases abundance of juvenile corals on a Caribbean reef". Proceedings of the National Academy of Sciences. 98 (9): 5067–5071. doi:10.1073/pnas.071524598. ISSN 0027-8424.
- ^ McClanahan, T.R.; Kaunda-Arara, B. (1996-08). "Fishery Recovery in a Coral-reef Marine Park and Its Effect on the Adjacent Fishery". Conservation Biology. 10 (4): 1187–1199. doi:10.1046/j.1523-1739.1996.10041187.x. ISSN 0888-8892.
{{cite journal}}
: Check date values in:|date=
(help) - ^ Sammarco, Paul W. (1980-01-01). "Diadema and its relationship to coral spat mortality: Grazing, competition, and biological disturbance". Journal of Experimental Marine Biology and Ecology. 45 (2): 245–272. doi:10.1016/0022-0981(80)90061-1. ISSN 0022-0981.
- ^ a b c d Page, Christopher A.; Muller, Erinn M.; Vaughan, David E. (2018-11-01). "Microfragmenting for the successful restoration of slow growing massive corals". Ecological Engineering. 123: 86–94. doi:10.1016/j.ecoleng.2018.08.017. ISSN 0925-8574.