User:SurjyenduB/sandbox/magnesite clumped
Clumped Isotope Geochemistry of Magnesite
[edit]The recent advancement in the field of stable isotope geochemistry is the study of isotopic structure of minerals and molecules. This requires study of molecules with high resolutions looking at not only substitution by heavier isotopes but also bonding scenario (how are they bonded to each other)- leading to knowledge of stability of molecule depending on its isotopic structure.
Isotopic Structure
[edit]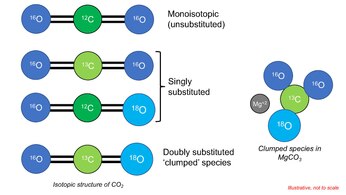
Oxygen has three stable isotopes (16O, 17O and 18O) and Carbon has two (13C, 12C). A 12C16O2 molecule (composed only with most abundant isotopes of constituent elements) is called 'monoisotopic' species. When only one atom is replaced with heavy isotope of any constituent element (ie, 13C16O2), it is called 'singly-substituted' species. Likewise, when two atoms are simultaneously replaced with heavier isotopes (eg., 13C16O18O), it is called 'doubly substituted' species. The 'clumped' species (13C16O18O) for CO2 is a doubly substituted CO2 molecule. Isotopically substituted molecules have higher mass. As a consequence, molecular vibration reduces, molecule develops lower zero point energy (see Kinetic isotope effect).
The abundances of certain bonds in certain molecules are sensitive to temperature at which it formed (e.g., abundance of 13C16O18O in carbonates[1] as 13C-18O bond). This information has been exploited to form the foundation of clumped isotope geochemistry and it is a rapidly developing field. Clumped isotope thermometer has been established for carbonate minerals like dolomite[2][3], calcite[4], siderite[5] etc and non-carbonate compounds like methane[6] and oxygen[7]. Depending on the strength of cation-carbonate oxygen (ie, Mg-O, Ca-O) bonds- different carbonate minerals can form or preserve clumped isotopic signature differently.
Isotopic Structure: Measurements and Reporting
[edit]There are some difficulties on employing regular methods of clumped isotopic analyses for magnesite. These are:
Digestion and Acid Fractionation Correction
[edit]Clumped isotopic analysis are usually done by gas source mass spectrometry where the CO2 liberated from acid digestion (reacting magnesite powder with phosphoric acid) of the carbonate is fed into the mass spectrometer. In such scenario, one needs to make sure that liberation of CO2 from magnesite is complete. Digesting magnesite is hard since it takes long time and different labs report different digestion time and temperature (from 12 hours at 100oC[8] to 1 hour at 90oC[9] in phosphoric acid). Due to digestion at this high temperature, some of the 13C-18O bonds in the liberated CO2 are broken (leading to reduction in abundance of 'clumped' CO2) during phosphoric acid digestion of carbonates. To account for these broken bonds (which is analytical artifact), a correction called 'acid fractionation correction' is added to the magnesite clumped isotope value obtained at temperature of digestion.
Δ47 - Temperature Calibration
[edit]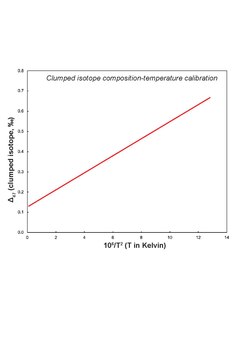
To convert clumped isotope data into temperature, a calibration curve is required which expresses the functional form of temperature dependence of clumped isotope composition. Such calibration exists for calcite[1], dolomite[3], siderite[5] as well as universal ones[10]. Different labs have their own calibrations which are substantiated through mineral precipitation experiments. Mineral specific calibrations are required owing to different acid digestion temperature and mineral behavior. This could be the case for magnesite as well since bonding is different than calcite/dolomite- changing mineral properties and requiring different calibration line. No mineral specific calibration exists for magnesite. But based on some experimental data[9] where experimental mineral precipitation temperature and clumped isotope derived temperatures don't match, a need of mineral specific calibration emerges.
Acid Fractionation Factor for O Isotope
[edit]Since the CO2 gas is liberated from carbonate mineral during acid digestion, leaving one O behind- a fractionation occurs, and the isotopic composition of the analyzed CO2 gas needs to be corrected for this. For magnesite, the most accurate fractionation factor(α) equation is given as[11]:
103ln(α) = [(6.845 ± 0.475)∗105/T2] + (4.22 ± 0.08); T in K
Different researchers have also used other fractionation factors like dolomite fractionation factor[12].
Standards
[edit]While measuring samples of unknown composition, it is required to measure some standard materials. This helps the user know (1) how the instrumental behavior is influencing the measurement (drift), if instrument prefers certain isotope over others (bias) and how these are affecting the analyses and (2) if the analyses are being conducted correctly and (3) to standardize the analysis. With internal standards and reference materials, analytical session is routinely monitored. Standard materials are majorly calcite and marble.
Magnesite-Water and CO2-Magnesite Isotope Fractionation Factors
[edit]Using temperature calibration, one can obtain temperature of formation of certain carbonate mineral using clumped isotope vaue. This knowledge enables one to know the C and O isotopic composition of the parental fluid using known fractionation factors, since fractionation (difference between isotopic composition of carbonate and fluid) is temperature dependent. Reported Magnesite-fluid and magnesite-CO2 O and C isotope fractionation factors in literature are not in agreement with each other[9]. The fractionation behaviors have not been substantiated by experimental observation- making this a bit problematic!
Current Understanding: Stable and Clumped Isotopes
[edit]Clumped isotopes have been used in interpreting conditions of magnesite formation and the isotopic composition of the precipitating fluid. Within ultramafic complexes, magnesites are found within veins and stockworks in cryptocrystalline form as well as within carbonated peridotite units in crystalline form. They form by carbonation of peridotites containing minerals like olivine and serpentine. The cryptocrystalline forms are mostly variably weathered and yield low clumped isotope derived temperature of formation[13]. On the other hand, coarse magnesites yield very high temperature indicating hydrothermal origin. It is speculated that coarse high temperature magnesites are formed from mantle derived fluids whereas cryptocrystalline ones are precipitated by circulating meteoric water- dictated by dissolved inorganic carbon pool, soil carbon and affected by disequilibrium isotope effects. Source fluid composition calculated from clumped isotope derived temperature indicates involvement of groundwater and organic carbon in forming cryptocrystalline magnesites.
Magnesites forming in lakes and playa settings are in general enriched in heavy isotopes of C and O because of evaporation and CO2 degassing. This reflects in the clumped isotope derived temperature being very low. These are affected by pH effect, biological activity as well as kinetic isotope effect associated with degassing and/or rapid CO2 uptake. Magnesite forms as surface moulds in such conditions but more generally occur as hydrous Mg-carbonates since their precipitation is kinetically favored.
Analysis of carbonates in Martian meteorite ALH84001 indicates that these formed at low temperature evaporative condition from subsurface water- deriving CO2 from Martian atmosphere[14].
Factors Controlling Clumped Isotope in Magnesite
[edit]
Mineral Structure and Later Thermal Effects
[edit]Crystalline and cryptocrystalline magnesites have very different mineral structures. While crystalline magnesite has a well developed crystal structure, the cryptocrystalline magnesite is amorphous- mostly aggregate of fine grained magnesite. Since clumped isotopic composition depends on specific bonding, difference in crystal structure is very likely to affect the way clumped isotopic signatures are recorded in these different structures. This leads to the fact that their pristine signatures might be modified differently by later thermal events like diagenesis/burial heating etc.
Disequilibrium
[edit]Disequilibrium processes like degassing, rapid CO2 uptake etc. modify clumped isotopic composition of carbonate minerals specifically at low temperatures. They variably enrich or deplete the system in heavy isotopes of C and O. Since clumped isotope abundance depends on abundance of isotopes of C and O, they are also modified. Another very prominent effect here is that of pH of precipitating fluid[15].
Presence of Co-genetic Carbonates
[edit]Often, magnesite records lower clumped isotope temperature than associated dolomite, calcite[16] which might be because of the fact that calcite, dolomite form earlier at higher temperature (from mantle like fluids) which increases Mg/Ca ratio in the fluid sufficiently so as to precipitate magnesite. As this happens with increasing time, fluid cools, evolves by mixing with other fluids and when it forms magnesite, it decreases its temperature. So the presence of associated carbonates have a control on magnesite isotopic composition.
Conversion from Hydrous Mg-Carbonates to Magnesite
[edit]Magnesite formation is kinetically hindered at low temperature due to solvation of Mg+2 ions. In low temperature, thus, hydrous Mg-carbonates (hydromagnesite, nesquehonite etc.) form. It is possible to convert these phases into magnesite by changing temperature by mineral dissolution-precipitation or heating. This dehydrates these hydrous phases. While so happens, an isotope effect associated can control the isotopic composition of precipitated magnesite.
Future Developments
[edit]Fixing of technical issues in this thermometer is one of the most important tasks to do. Identification of carbonates- specifically Mg-carbonate lithologies on Mars has opened up new avenues about our understanding of Martian geology. Lake condition has been reported to have prevailed near Jezero crater[17]. Origin of these carbonates can be deconvolved with the possible application of clumped isotope thermometer and as well as source of the CO2, climatic-hydrologic conditions on Mars could be assessed from these rocks.
References
[edit]- ^ a b Ghosh, Prosenjit; Adkins, Jess; Affek, Hagit; Balta, Brian; Guo, Weifu; Schauble, Edwin A.; Schrag, Dan; Eiler, John M. (2006-03-15). "13C–18O bonds in carbonate minerals: A new kind of paleothermometer". Geochimica et Cosmochimica Acta. 70 (6): 1439–1456. doi:10.1016/j.gca.2005.11.014. ISSN 0016-7037.
- ^ Lloyd, Max K.; Ryb, Uri; Eiler, John M. (2018-12-01). "Experimental calibration of clumped isotope reordering in dolomite". Geochimica et Cosmochimica Acta. 242: 1–20. doi:10.1016/j.gca.2018.08.036. ISSN 0016-7037.
- ^ a b Winkelstern, Ian Z.; Kaczmarek, Stephen E.; Lohmann, Kyger C; Humphrey, John D. (2016-12-02). "Calibration of dolomite clumped isotope thermometry". Chemical Geology. 443: 32–38. doi:10.1016/j.chemgeo.2016.09.021. ISSN 0009-2541.
- ^ Stolper, D. A.; Eiler, J. M. (2015-05-01). "The kinetics of solid-state isotope-exchange reactions for clumped isotopes: A study of inorganic calcites and apatites from natural and experimental samples". American Journal of Science. 315 (5): 363–411. doi:10.2475/05.2015.01. ISSN 0002-9599.
- ^ a b van Dijk, Joep; Fernandez, Alvaro; Storck, Julian C.; White, Timothy S.; Lever, Mark; Müller, Inigo A.; Bishop, Stewart; Seifert, Reto F.; Driese, Steven G.; Krylov, Alexey; Ludvigson, Gregory A. (2019-06). "Experimental calibration of clumped isotopes in siderite between 8.5 and 62 °C and its application as paleo-thermometer in paleosols". Geochimica et Cosmochimica Acta. 254: 1–20. doi:10.1016/j.gca.2019.03.018. ISSN 0016-7037.
{{cite journal}}
: Check date values in:|date=
(help) - ^ Stolper, D. A.; Lawson, M.; Davis, C. L.; Ferreira, A. A.; Neto, E. V. Santos; Ellis, G. S.; Lewan, M. D.; Martini, A. M.; Tang, Y.; Schoell, M.; Sessions, A. L. (2014-06-27). "Formation temperatures of thermogenic and biogenic methane". Science. 344 (6191): 1500–1503. doi:10.1126/science.1254509. ISSN 0036-8075. PMID 24970083.
- ^ Yeung, Laurence Y.; Young, Edward D.; Schauble, Edwin A. (2012). "Measurements of 18O18O and 17O18O in the atmosphere and the role of isotope-exchange reactions". Journal of Geophysical Research: Atmospheres. 117 (D18). doi:10.1029/2012JD017992. ISSN 2156-2202.
- ^ Śliwiński, Maciej G.; Kitajima, Kouki; Spicuzza, Michael J.; Orland, Ian J.; Ishida, Akizumi; Fournelle, John H.; Valley, John W. (2017-11-22). "SIMS Bias on Isotope Ratios in Ca-Mg-Fe Carbonates (Part III): δ18O and δ13C Matrix Effects Along the Magnesite-Siderite Solid-Solution Series". Geostandards and Geoanalytical Research. 42 (1): 49–76. doi:10.1111/ggr.12194. ISSN 1639-4488.
- ^ a b c García del Real, Pablo; Maher, Kate; Kluge, Tobias; Bird, Dennis K.; Brown, Gordon E.; John, Cédric M. (2016-11). "Clumped-isotope thermometry of magnesium carbonates in ultramafic rocks". Geochimica et Cosmochimica Acta. 193: 222–250. doi:10.1016/j.gca.2016.08.003. ISSN 0016-7037.
{{cite journal}}
: Check date values in:|date=
(help) - ^ Bonifacie, Magali; Calmels, Damien; Eiler, John M.; Horita, Juske; Chaduteau, Carine; Vasconcelos, Crisogono; Agrinier, Pierre; Katz, Amandine; Passey, Benjamin H.; Ferry, John M.; Bourrand, Jean-Jacques (2017-03). "Calibration of the dolomite clumped isotope thermometer from 25 to 350 °C, and implications for a universal calibration for all (Ca, Mg, Fe)CO3 carbonates". Geochimica et Cosmochimica Acta. 200: 255–279. doi:10.1016/j.gca.2016.11.028. ISSN 0016-7037.
{{cite journal}}
: Check date values in:|date=
(help) - ^ Sharma, S.Das; Patil, D.J; Gopalan, K (2002-02). "Temperature dependence of oxygen isotope fractionation of CO 2 from magnesite-phosphoric acid reaction". Geochimica et Cosmochimica Acta. 66 (4): 589–593. doi:10.1016/s0016-7037(01)00833-x. ISSN 0016-7037.
{{cite journal}}
: Check date values in:|date=
(help) - ^ Rosenbaum, J; Sheppard, S.M.F (1986-06). "An isotopic study of siderites, dolomites and ankerites at high temperatures". Geochimica et Cosmochimica Acta. 50 (6): 1147–1150. doi:10.1016/0016-7037(86)90396-0. ISSN 0016-7037.
{{cite journal}}
: Check date values in:|date=
(help) - ^ Quesnel, Benoît; Boulvais, Philippe; Gautier, Pierre; Cathelineau, Michel; John, Cédric M.; Dierick, Malorie; Agrinier, Pierre; Drouillet, Maxime (2016-06). "Paired stable isotopes (O, C) and clumped isotope thermometry of magnesite and silica veins in the New Caledonia Peridotite Nappe". Geochimica et Cosmochimica Acta. 183: 234–249. doi:10.1016/j.gca.2016.03.021. ISSN 0016-7037.
{{cite journal}}
: Check date values in:|date=
(help) - ^ Halevy, Itay; Fischer, Woodward W.; Eiler, John M. (2011-10-11). "Carbonates in the Martian meteorite Allan Hills 84001 formed at 18 ± 4 °C in a near-surface aqueous environment". Proceedings of the National Academy of Sciences. 108 (41): 16895–16899. doi:10.1073/pnas.1109444108. ISSN 0027-8424. PMC 3193235. PMID 21969543.
{{cite journal}}
: CS1 maint: PMC format (link) - ^ Guo, Weifu (2020-01). "Kinetic clumped isotope fractionation in the DIC-H2O-CO2 system: Patterns, controls, and implications". Geochimica et Cosmochimica Acta. 268: 230–257. doi:10.1016/j.gca.2019.07.055. ISSN 0016-7037.
{{cite journal}}
: Check date values in:|date=
(help) - ^ Streit, Elisabeth; Kelemen, Peter; Eiler, John (2012-06-17). "Coexisting serpentine and quartz from carbonate-bearing serpentinized peridotite in the Samail Ophiolite, Oman". Contributions to Mineralogy and Petrology. 164 (5): 821–837. doi:10.1007/s00410-012-0775-z. ISSN 0010-7999.
- ^ Horgan, Briony H.N.; Anderson, Ryan B.; Dromart, Gilles; Amador, Elena S.; Rice, Melissa S. (2020-03). "The mineral diversity of Jezero crater: Evidence for possible lacustrine carbonates on Mars". Icarus. 339: 113526. doi:10.1016/j.icarus.2019.113526. ISSN 0019-1035.
{{cite journal}}
: Check date values in:|date=
(help)