User:Sadalsuud/Sandbox
Towards FAC
[edit]Orion Mouseover
[edit]Angular size rework
[edit]Calculations
[edit]Click on the "[show]" link in the box below.
Van Leeuwen distance
van Leeuwen 2007 distance of 152 ± 20 pc.[1]
Luminosity Calculations
Luminosity Calculations[2] EndLuminosityCalcs
The following calculations determine Betelgeuse's average radius in terms of solar units based on the following assumptions for angular diameter:
1) Weiner 2000 = 55.20 ± 0.50 mas (limb darkened)
2) Perrin 2004 = 43.33 ± 0.04 mas
The calculations begin with the formula for a star's angular diameter, as follows:
where
represents Betelgeuse's angular diameter in arcseconds,
the Distance from Earth in parsecs
Betelgeuse's diameter in AU, and
Betelgeuse's Radius in AU. Therefore:
- (rounded)
- (rounded)
To convert the above into Solar units, the math is straightforward. Since 1 AU = 149,597,871 km and the mean diameter of the Sun = 1,392,000 km (hence a mean radius of 696,000 km), the calculation is as follows:
- (rounded).
- (rounded).
Luminosity Note
Nevertheless, since Betelgeuse is a pulsating variable star, there are times where the star's luminosity could theoretically exceed 200,000L☉[note 1]
- where... B = Betelgeuse, L = Luminosity, R = Radius and T = Temperature.
Therefore:
Note: These calculations do not take into consideration any diminution caused by extinction, which in the case of Betelgeuse has been estimated at around 3.1%
Text
[edit]The current debate revolves around which wavelength—the visible, near-infrared (NIR) or mid-infrared (MIR)—produces the most accurate angular measurement.[note 2] In 1996, Manfred Bester,working with the ISI in the mid-infrared, led a team at the Space Sciences Laboratory (SSL) at U.C. Berkeley to produce a solution showing Betelgeuse with a uniform disk of 56.6 ± 1.0 mas. In 2000, the SSL team produced another measure of 54.7 ± 0.3 mas, ignoring any possible contribution from hotspots, which are less noticeable in the mid-infrared.[3] Also included was a theoretical allowance for limb darkening yielding a diameter of 55.2 ± 0.5 mas. The Bester estimate equates to a radius of roughly 5.6 AU or 1,200 R☉, assuming the 2008 Harper distance of 197.0 ± 45 pc,[4] a figure roughly the size of the Jovian orbit of 5.5AU, published in 2009 in Astronomy Magazine and a year later in NASA's Astronomy Picture of the Day.[5]
Across the Atlantic, another team of astronomers working in the near-infrared and led by Guy Perrin of the Observatoire de Paris produced a 2004 document arguing that the more accurate photospheric measurement was 43.33 ± 0.04 mas.[6] The study also put forth an explanation as to why varying wavelengths from the visible to mid-infrared produce different diameters. The star is seen through a thick, warm extended atmosphere. At short wavelengths (the visible spectrum) the atmosphere scatters light thus slightly increasing the star's diameter. At near-infrared wavelengths (K and L bands), the scattering is negligible, so the classical photosphere can be directly seen; in the mid-infrared the scattering increases once more causing the thermal emission of the warm atmosphere to increase the apparent diameter.[6] Comparing studies, however, is problematic since each wavelength produces a different view of the star, requiring different interpretations.[7]

Studies done in 2009 with the IOTA and VLTI brought strong support to Perrin's analysis yielding diameters ranging from 42.57 to 44.28 mas with comparatively insignificant margins of error.[9][10] In 2011, Keiichi Ohnaka from the Max Planck Institute for Radio Astronomy produced a third estimate in the near-infrared corroborating Perrin's numbers, this time showing a limb-darkened disk diameter of 42.49 ± 0.06 mas.[11] Consequently, if we combine the smaller Hipparcos distance from van Leeuwen of 152 ± 20 pc with Perrin's angular measurement of 43.33 mas, a near-infrared photospheric estimate would equate to 3.4AU or roughly 730R☉.[12]
Central to this discussion is another paper published by the Berkeley team in 2009, this time led by Charles Townes, reporting that the radius of Betelgeuse had actually shrunk from 1993 to 2009 by 15%, with the 2008 angular measurement equal to 47.0 mas, not too far from Perrin's estimate.[13][14] Unlike most papers heretofore published, this study encompassed a 15-year period at one specific wavelength. Earlier studies have typically lasted one to two years by comparison and have explored multiple wavelengths, often yielding vastly different results. The diminution in Betelgeuse's apparent size equates to a range of values between 56.0 ± 0.1 mas seen in 1993 to 47.0 ± 0.1 mas seen in 2008—a contraction of almost 0.9 AU in 15 years. What is not fully known is whether this observation is evidence of a rhythmic expansion and contraction of the star's photosphere as astronomers have theorized, and if so, what the periodic cycle might be, although Townes suggested that if a cycle does exist, it is probably a few decades long.[13] Other possible explanations are photospheric protrusions due to convection or a star that is not spherical but rather asymmetric causing the appearance of expansion and contraction as the star rotates on its axis.[15]
In conclusion, the current debate between measurements in the mid-infrared, which suggest a possible expansion and contraction of the star, versus the relatively constant photosphere observed in the near-infrared is yet to be resolved. In a recent paper published in 2012, however, the Berkeley team reported that their measurements were "dominated by the behaviour of cool, optically thick material above the stellar photosphere", indicating that the apparent expansion and contraction may be due to activity in the star's outer shells and not the photosphere itself. This conclusion, if further corroborated, would suggest an average angular diameter for Betelgeuse closer to Perrin's estimate at 43.33 arcseconds, hence a stellar radius of about 3.4 AU (730R☉), if we assume the shorter Hipparcos distance of 498±73ly in lieu of Harper's estimate at 643±146ly.[note 3][16] The upcoming Gaia Mission will likely clarify many of the assumptions presently used in calculating the size of Betelgeuse's stellar disk, yielding a much wider consensus among astronomers.
Once considered as having the largest angular diameter of any star in the sky after the Sun, Betelgeuse lost that distinction in 1997 when a group of astronomers measured R Doradus with a diameter of 57.0 ± 0.5 mas. Betelgeuse is now considered to be in third place, although R Doradus, being much closer to Earth at about 200 ly, has an actual diameter roughly one-third that of Betelgeuse.[17]
Star system rework
[edit]In 1985, Margarita Karovska, in conjunction with other astrophysicists at the CfA, announced the discovery of two close companions orbiting Betelgeuse. Analysis of polarization data from 1968 through 1983 indicated a close companion with a periodic orbit of about 2.1 years. Using speckle interferometry, the team concluded that the closer of the two companions was located at 0.06 ± 0.01" (~9 AU) from the main star with a position angle (PA) of 273 degrees, an orbit that would potentially place it within the star's chromosphere. The more distant companion was estimated at 0.51 ± 0.01" (~77 AU) with a PA of 278 degrees.[18][19]
In the years that followed no confirmation of Karovska's discovery was ever published. Rather in 1992, a team of collaborators from the Cavendish Astrophysics Group published a paper noting that the brightness features on the surface of Betelgeuse appear to be "too bright to be associated with a passage of the suggested companions in front of the red giant." They also noticed that these features were fainter at 710 nanometers compared to 700 by a factor of 1.8, indicating that such features would have to reside within the molecular atmosphere of the star.[20]
That same year, Karovska published a new paper reconfirming her team's exegesis, but also noting that there was a meaningful correlation between the calculated position angles of the orbiting companion and the reported asymmetries, suggesting a possible connection between the two.[21] Since then, researchers have turned their attention to analyzing the intricate dynamics of the star's extended atmosphere and little else has been published on the possibility of orbiting companions, although as Xavier Haubois and his team reiterate in 2009, the possibility of a close companion contributing to the overall flux has never been fully ruled out.[10] Dommanget's double star catalog (CCDM) lists at least four adjacent stars, all within three arcminutes of this stellar giant, yet aside from apparent magnitudes and position angles, little else is known.[22] As the decade unfolds and new technologies are brought to unraveling the star's enigmatic past, conclusive evidence will likely emerge of any potential star system. Given the planned capabilities of the upcoming Gaia mission, a confirmation could occur any time after the mission's scheduled launch in August 2013.[23]
Betelgeuse Mass Rework
[edit]Because the original discovery of a companion star in 1985 by Karovska was never confirmed, there is no direct method of measuring Betelgeuse's mass.[24] A mass estimate is only possible using theoretical modeling, a situation which has produced mass estimates ranging from 5—30M☉ in the last decade.[25] [26] The current estimate that is widely accepted is 17.5±2.5M⊙, based on a photospheric measurement of 5.6AU or 1,200R⊙. However, a novel method of determining the supergiant's mass was proposed in 2011 by Hilding Neilson et al. arguing for a stellar mass of 11.6M⊙, based on observations of the star’s intensity profile from narrow H-band interferometry and using a photospheric measurement of 4.3AU or 995R⊙. How the debate is resolved is still open to question—at least until a companion star is identified allowing for a direct calculation of the star's mass.
These uncertainties also mean that no consensus has yet emerged regarding the star's mass. Estimates range from 5 to 30 solar masses (M☉) with most investigators showing a preference for a relatively large mass ranging from 10 to 20M☉. One model reports a mass at the lower end of the scale at 14M☉, although a mass ranging from 18 to 20 is more commonplace.[25][27]
Comment
[edit]- "most investigators showing a preference for a relatively large mass ranging from 10 to 20M☉. One model reports a mass at the lower end of the scale at 14M☉, although a mass ranging from 18 to 20 is more commonplace". Which is the commoner range? 10 to 20, or 18 to 20? These appear inconsistent. hamiltonstone (talk) 04:38, 4 September 2012 (UTC)
- Need to research This section was originally drafted in 2010. But Mohamed 2012 may have the answer. I'll research this and update accordingly--Sadalsuud (talk) 15:50, 7 September 2012 (UTC)
- Research done Unfortunately, Mohamed2012 doesn't provide any additional clarity. So we are faced with a judgment call as editors. On page 2 of Mohamed 2012, the authors put forth a handy table of basic stellar paramaters. The first on the list is Mass. But instead of resolving the debate, Mohamed quotes two distinct papers with vastly different parameters, one from Neilson 2011 showing a Mass = 11.6+5.0−3.9M⊙, the second from Smith 2009 showing Mass = 15—20M⊙, which if you were to combine the two would yield a range where Mass ≈ 7.7—20M⊙—clearly not a very good solution for our purposes.
- When you study the two underlying papers, however, you notice something interesting. The Neilson2011 document has Haubois as a co-author. Haubois is one of the astronomers who has been working with Perrin and Kervella using the VLT in Chile and arguing that a near-infrared diameter is the more accurate photosperic measurement. So the 11.6M⊙is based on the smaller photospheric measurement of 4.3AU or 995R⊙. Similarly, the 17.5±2.5M⊙is based on the research being done by the Berkeley team and is based on a photospheric measurement of 5.6AU or 1,200R⊙.
- Seeing this, I have gone ahead and edited the section on Mass, carrying forward the same theme found at the conclusion of the Angular size discussion. Since 5.5AU is still the de facto standard, I have chosen 17.5±2.5M as the standard Mass, while hinting at the Mass being considerably smaller, should consensus move in the direction of a smaller photospheric measurement. Instead of using a range as Mohamed and Smith do, chosing the midpoint of 17.5 gives us the simplicity we are looking for.--Sadalsuud (talk) 17:47, 9 September 2012 (UTC)
- It's a challenging issue, no question. As you have already surmised, the problem comes down to a lack of consensus on two issues: 1) distance and 2) angular diameter. I've googled magazine articles, but they are either vague or understandably non-committal: Astronomy Magazine, Astronomy Now, and Bad Astronomy. Primary sources will take a position on either distance or angular diameter. Mohamed2012 as recently as May 2012, published a paper using Smith2009 who quotes Bester1996 establishing a radius of 5.6 AU or 1,200 Solar, based on Harper2008 figure of 197±45 pc. I suspect that the APOD2010 pic as well as the AAVSO and Astronomy Magazine articles use that source as they were all published just after.
FAC Rework
[edit]Across the Atlantic, another team of astronomers led by Guy Perrin of the Observatoire de Paris produced a document in 2004 arguing that the near-infrared figure of 43.33 ± 0.04 mas was a more accurate photospheric measurement.[6] "A consistent scenario to explain the observations of this star from the visible to the mid-infrared can be set-up", Perrin reports. "The star is seen through a thick, warm extended atmosphere that scatters light at short wavelengths thus slightly increasing its diameter. The scatter becomes negligible above 1.3 μm. The upper atmosphere being almost transparent in K and L—the diameter is minimum at these wavelengths where the classical photosphere can be directly seen. In the mid-infrared, the thermal emission of the warm atmosphere increases the apparent diameter of the star." The argument has yet to receive widespread support among astronomers.[7]
Across the Atlantic, another team of astronomers led by Guy Perrin of the Observatoire de Paris produced a document in 2004 arguing that the near-infrared figure of 43.33 ± 0.04 mas was a more accurate photospheric measurement.[6] The study also puts forth an explanation as to why varying wavelengths from the visible to mid-infrared produce different diameters. The star is seen through a thick, warm extended atmosphere. At short wavelengths (the visible spectrum) the atmosphere scatters light thus slightly increasing its diameter. At infrared wavelengths (K and L bands), the scattering is negligible, so the classical photosphere can be directly seen; in the mid-infrared the scattering increases once more causing the thermal emission of the warm atmosphere to increase the apparent diameter.[6] Comparing studies at different wavelengths, however, is problematic, since each wavelength produces a different view of the star, requiring different interpretations.[7]
I reworked paragraph pursuant to observations
Density Calculations
[edit]To compute these density ratios, the first step is to calculate the mass and volume of each component from which density calculations can be made. Given the density of the Sun, Betelgeuse, and the Earth's atmosphere, dividing gives the appropriate ratios.
Calculations for the Sun:
- Solar mass ≈ 1.9891×1030 kg
- Solar volume ≈ 1.412 × 1018 km3 × 109 (to convert to m3) ≈ 1.412 × 1027 m3
- Solar density ≈ 1.9891×1030 kg ÷ 1.412 × 1027 m3 ≈ 1.409 × 103 kg/m3.
Calculations for Betelgeuse:
- Betelgeuse mass ≈ 18.5 x Solar × 1.9891×1030 ≈ 3.680 ×1031 kg
- Betelgeuse volume ≈ 2.335 × 1027 km3 × 109 (to convert to m3) ≈ 2.335 × 1036 m3 (i.e. 5.5AU)
- Betelgeuse density ≈ 3.680 ×1031 kg ÷ 2.335 × 1036 m3 ≈ 1.576 × 10−5 kg/m3.
Calculations for the Earth's atmosphere:
- Atmospheric Mass ≈ 5.148 × 1018 kg
- Atmospheric volume ≈ 4.200 × 1009 km3 × 109 (to convert to m3) ≈ 4.200 × 1018 m3
- Atmospheric Density ≈ 5.148 × 1018 kg ÷ 4.200 × 1018 m3 ≈ 1.226 kg/m3.
Average Density Ratios:
- Betelgeuse/Sun ≈ 1.576 × 10−5 kg/m3 ÷ 1.409 × 103 kg/m3 ≈ 1.119 × 10−8
- Betelgeuse/Earth's Atmosphere = 1.576 × 10−5 kg/m3 ÷ 1.226 kg/m3 ≈ 1.286 × 10−5
Conclusion:
- Earth's Atmospheric Density at various altitudes is graphically represented by the NRLMSISE-00 atmospheric model. The average atmospheric density for Betelgeuse is 1.576 × 10−5 kg/m3 or 1.576 × 10−8 g/cm3, which, compared to the model, places it somewhere between 90 and 95 km above sea level relative to Earth. Noctilucent clouds are found between 76 and 85 km above sea level.</ref> Such star matter is so tenuous, in fact, that Betelgeuse has often been called a "red-hot vacuum".[28][29]
Notes Rework
[edit]The following calculations determine Betelgeuse's average radius in terms of solar units based on the following assumptions for angular diameter:
1) Weiner 2000 = 55.20 ± 0.50 mas (limb darkened)
2) Perrin 2004 = 43.33 ± 0.04 mas
The calculations begin with the formula for a star's angular diameter, as follows:
where
represents Betelgeuse's angular diameter in arcseconds,
the Distance from Earth in parsecs
Betelgeuse's diameter in AU, and
Betelgeuse's Radius in AU. Therefore:
- (rounded)
- (rounded)
To convert the above into Solar units, the math is straightforward. Since 1 AU = 149,597,871 km and the mean diameter of the Sun = 1,392,000 km (hence a mean radius of 696,000 km), the calculation is as follows:
- (rounded).
- (rounded).
Luminosity article
[edit]Luminosity is generally understood as a measurement of brightness. Each discipline, however, defines the term differently, depending on what is being measured.
In astronomy, luminosity measures the total amount of energy emitted by a star or other astronomical object in joules per second, which are watts. Watts are the unit of power, and so just as a light bulb is measured in watts, so too is the Sun, the latter having a total power output of 3.846×1026 W. It is this number which constitutes the basic metric used in astronomy and is known as 1 solar luminosity, the symbol for which is . Radiant power, however, is not the only way to conceptualize brightness, so other metrics are also used. The most common is apparent magnitude, which is the perceived brightness of an object from an observer on Earth at visible wavelengths. Other metrics are absolute magnitude, which is an object's intrinsic brightness at visible wavelengths, irrespective of distance, while bolometric magnitude is the total power output across all wavelengths.
The field of optical photometry uses a different set of distinctions, the main ones being luminance and illuminance. Astronomical photometry, by contrast, is concerned with measuring the flux, or intensity of an astronomical object's electromagnetic radiation. In the field of computer graphics the concept of luminosity is different altogether and is typically used as a synonym for the concept of lightness, otherwise known as the value or tone component of a color.
Astronomy
[edit]In astronomy, luminosity is the amount of electromagnetic energy a body radiates per unit of time. It is measured in two forms: apparent (visible light only) and bolometric (total radiant energy). A bolometer is the instrument used to measure radiant energy over a wide band by absorption and measurement of heating. When not qualified, the term "luminosity" means bolometric luminosity, which is measured either in the SI units, watts, or in terms of solar luminosities. A star also radiates neutrinos, which carry off some energy, about 2% in case of our sun, producing a stellar wind and contributing to the star's total luminosity.[citation needed]
A star's luminosity is determined primarily by two stellar characteristics: size and temperature. The former is typically represented in terms of solar radii, , while the latter is represented in degrees Kelvin. To determine a star's radius, however, two other metrics are needed: the star's angular diameter and its distance from Earth, usually calculated using parallax measurements. A third metric is the degree of interstellar extinction that is present, a condition that usually arises because of gas and dust present in the interstellar medium (ISM), the Earth's atmosphere, and circumstellar matter. Consequently, one of astronomy's central challenges in determining a star's luminosity is to derive accurate measurements for each of these components, without which an accurate luminosity figure remains elusive.
In the current system of stellar classification, stars are grouped according to temperature, with the very young and energetic Class O stars boasting temperatures in excess of 30,000K while the older Class M stars exhibit temperatures less than 3,700K—a vast difference that has a huge impact on the star's luminosity. As a result, the most luminous stars in the galaxy are usually the youngest; as stars evolve, their stellar wind causes them to lose mass, a phenomenon which varies greatly with each class of stars but which nevertheless causes a diminution in the star's luminosity over its lifetime. In the Hertzsprung-Russel diagram, the vast majority of stars are found along the main sequence with blue Class 0 stars found at the top left of the chart, given their high luminosity, while red Class M stars fall to the right. The reason certain stars like Deneb and Betelgeuse are found off the main sequence is because of their extremely high luminosity resulting from their enormous size. A star like Deneb, for instance, has a radius that is 203, yielding a mass of 19 and luminosity of 196,000, which means that this blue-white supergiant radiates one hundred and ninety-six thousand times as much energy as the Sun.[30] By contrast, the much cooler Betelgeuse has a luminosity of approximately 140,000, a figure which is only possible because it is considerably larger than Deneb; with a radius of 995, Betelgeuse is about 15 times its size. The most brilliant star ever discovered is a Wolf-Rayet star known as R136a1, spotted in the Large Magellanic Cloud in 2010. At 265 it is one of the most massive and most luminous stars yet identified, boasting a total bolometric luminosity 8,700,000 times that of the Sun.
To conceptualize the range of magnitudes in our own galaxy, the Milky Way, the smallest star to be identified has about 8% of the Sun’s mass and glows feebly at absolute magnitude +19. Compared to the Sun, which has an absolute of +4.8, this faint star is 14 magnitudes or 400,000 times dimmer than our Sun. Our galaxy's most massive stars begin their lives with masses of roughly 100 times solar, radiating at upwards of absolute magnitude –8, over 160,000 times the solar luminosity. The total range of stellar luminosities, then, occupies a range of 27 magnitudes, or a factor of 60 billion!
Miscellaneous text
[edit]The most common distinctions encountered are apparent magnitude, which is the measurement of a celestial body's visible light as seen by an observer from Earth. By contrast, absolute Magnitude is a visible light measurement irrespective of distance and so measures a luminous body's intrinsic brightness. Finally, bolometric magnitude measures light at all wavelengths in the electromagnetic spectrum. Every star will thus have three different measurements. Betelgeuse has an median apparent magnitude of 0.42, but given a distance of 197 parsecs has an absolute magnitude of -6.02. Being a cool, red star, its bolometric luminosity is -7.99, which means the visible light that reaches our eye is only 6% of the star's total radiant energy. If the human eye could see light at all wavelengths, Betelgeuse would show up as the brightest star in the sky.
The word "luminosity" may also refer to spectral luminosity, measured either in W/Hz or W/nm.
Betelgeuse article
[edit]Circumstellar Rework
[edit]Old intro
[edit]In the late phase of stellar evolution, massive stars like Betelgeuse exhibit high rates of mass loss, possibly as much as 1M☉ every 10,000 years, resulting in a complex circumstellar environment that is constantly in flux.[31] All stars exhibit mass loss. Rates vary from about 10−14 to 10−4 yr −1 depending on spectral type, luminosity class, rotation rate, companion proximity, and evolutionary stage.[32] Exactly how this mass loss occurs, however, has been a mystery confronting astronomers for decades. When Schwarzschild first proposed his theory of monster convection cells, he argued it was the likely cause in red supergiants. Prior attempts to explain mass loss in terms of solar wind theory had proven unsuccessful as they led to a contradiction with observations involving circumstellar shells.[33] Other theories that have been advanced include magnetic activity, global pulsations and shock structures as well as stellar rotation.[34]

As a result of work done by Pierre Kervella and his team at the Paris observatory in 2009, astronomers may be close to solving this mystery. Kervella noticed a large plume of gas extending outward at least six times the stellar radius indicating that the star is not shedding matter evenly in all directions.[35][31] The plume's presence, in fact, implies that the spherical symmetry of its photosphere, often observed in the infrared, is not preserved in its close environment. Asymmetries on the stellar disk had been reported many times at different wavelengths. However, due to the refined capabilities of the NACO adaptive optics on the VLT, these asymmetries have come into focus. The two mechanisms that could cause such asymmetrical mass loss, Kervella noted, were large-scale convection cells or polar mass loss, possibly due to rotation.[35] Probing deeper still with ESO's AMBER, Keiichi Ohnaka observed that the gas in the supergiant's extended atmosphere is vigorously moving up and down, creating bubbles as large as the supergiant itself, leading his team to conclude that such stellar upheaval is behind the massive plume ejection observed by Kervella.[31][36]
Asymmetric shells
[edit]In addition to the photosphere, six other components of Betelgeuse's atmosphere have now been identified. Extending outward, one encounters a molecular environment otherwise known as the MOLsphere, a gaseous envelope, a chromosphere, a dust environment and two outer shells (S1 and S2) composed of carbon monoxide (CO). There is also evidence of coronal plasma in the star's extended atmosphere, a phenomenon that heretofore was not believed to exist in late stage stars off the main sequence.[25] Some of these elements are known to be asymmetric while others overlap.[10]

At about .45 stellar radii (~2–3 AU) above the photosphere, the closest membrane appears to be the molecular layer known as the MOLsphere. Studies show it to be composed of water-vapor and carbon monoxide with an effective temperature of about 1500 ± 500K.[10][37] Water-vapor had been originally detected in the supergiant's spectrum back in the 1960s with the two Stratoscope projects but had been ignored for decades. Recent studies suggest that the MOLsphere may also contain SiO and Al2O3—molecules which could explain the formation of dust particles.

Between two and seven stellar radii (~10–40 AU), astronomers have identified another region known as an asymmetric gaseous envelope composed of elemental abundances C, N and O.[10][38] The radio-telescope images taken in 1998 confirm that Betelgeuse has a dense atmosphere with a "remarkably complex structure".[39] Observations show the atmosphere to be boiling with a temperature of 3,450 ± 850K—similar to the temperature recorded on the star's surface but much lower than surrounding gas in the same region.[39][40] The VLA images also showed this lower-temperature gas progressively decreasing in temperature as it extends outward—the existence of which, although unexpected, turns out to be the most abundant constituent of Betelgeuse's atmosphere. "This alters our basic understanding of red-supergiant star atmospheres", explained Jeremy Lim, the team's leader. "Instead of the star's atmosphere expanding uniformly because of gas heated to very high temperatures near its surface, it now appears that several giant convection cells propel gas from the star's surface into its atmosphere."[39] This is the same region in which Kervella's 2009 finding of a bright plume, possibly containing CN and extending at least six photospheric radii in the southwest direction of the star, is believed to exist.[10]
The chromosphere, as mentioned earlier, was resolved in 1996 at about 2.2 times the optical disk (~10 AU) at ultraviolet wavelengths and is reported to have a temperature no higher than 5,500K.[41][10] The image was taken with the Faint Object Camera on board the Hubble Space Telescope and also revealed a bright area in the southwest quadrant of the disk. However, in 2004 observations with the STIS, Hubble's high-precision spectrometer, pointed to the existence of warm chromospheric plasma at least one arcsecond away from the star. At a distance of 197 pc, the size of the chromosphere could be 200 AU[42] The CfA team led by Alex Lobel concluded that the spatially resolved STIS spectra directly demonstrate the co-existence of warm chromospheric plasma with cool gas in Betelgeuse's circumstellar dust envelope.[42]

The first attestation of a dust shell surrounding Betelgeuse was put forth by Sutton and colleagues, who noted in 1977 that dust shells around mature stars often emit large amounts of radiation in excess of the photospheric contribution. Using heterodyne interferometry, they concluded that the red supergiant emits most of its excess beyond 12 stellar radii or roughly the distance of the Kuiper belt at 50 to 60 AU, depending on the assumed stellar radius.[10][45] Since then, there have been numerous studies done of this dust envelope at varying wavelengths yielding decidedly different results. More recent studies have estimated the inner radius of the dust shell anywhere from 0.5 to 1.0 arcseconds, or 100 to 200 AU.[46][47] What these studies point out is that the dust environment surrounding Betelgeuse is anything but static. In 1994, Danchi et al. reported that Betelgeuse undergoes sporadic dust production involving decades of activity followed by inactivity. A few years later, a group of astronomers led by Chris Skinner noticed significant changes in the dust shell's morphology in just one year, suggesting that the shell is asymmetrically illuminated by a stellar radiation field strongly affected by the existence of photospheric hotspots.[46] The 1984 report of a giant asymmetric dust shell located 1 pc (206,265 AU) from the star has not been corroborated in recent studies, although another report published the same year said that three dust shells were found extending four light years from one side of the decaying star, suggesting that, like a snake, Betelgeuse sheds its outer layers as it journeys across the heavens.[48][49]
Although the exact size of the two outer CO shells remains elusive, preliminary estimates suggest that one shell extends from about 1.5 to 4.0 arcseconds with the other expanding as far as 7.0 arcseconds.[50] Using the Jovian orbit of 5.5 AU as the "average" radius for this gargantuan star, the inner shell would extend roughly 50 to 150 stellar radii (~300 to 800 AU) with the outer one as far as 250 stellar radii (~1400 AU). With the heliopause estimated at about 100 AU, the size of this outer shell is almost fourteen times the size of the Solar System.
Supersonic bow shock
[edit]Studies since the beginning of the millennium have revealed that Betelgeuse is travelling supersonically through the interstellar medium (ISM) at a speed of 30 km per second (i.e. ~6.3 AU per year) creating a bow shock.[51] The shock is not created by the star itself, but rather a powerful stellar wind as it ejects vast amounts of gas into the ISM at a rate of 17 km/sec, heating up the material surrounding the star thereby making it visible in infrared light. Because Betelgeuse is so bright, it was only in 1997 that the bow shock was first imaged. The cometary structure is estimated to be at least 1 parsec, assuming a current distance of 643 light years.[52]
Recent 3D hydrodynamic simulations of the bow shock indicate that it is very young—less than 30,000 years old—suggesting either of two possibilities: one, that Betelgeuse moved into a region of the ISM with very different properties recently or two, that Betelgeuse itself has undergone a significant transformation as its stellar wind has changed.[53] In their 2012 paper, Mohammed et al. propose that this phenomenon was caused by Betelgeuse transitioning from a blue supergiant (BSG) to a red supergiant (RSG). In fact, in the late evolutionary stage of a star like Betelgeuse, evidence suggests that stars "may undergo rapid transitions from red to blue and vice versa on the Hertzsprung-Russell diagram, with accompanying rapid changes to their stellar winds and bow shocks."[54][55] Moreover, if future research bears out this hypothesis, Betelgeuse may well prove to have traveled close to 200,000 AU as a red supergiant scattering as much as 3 along its trajectory.
Observational history
[edit]Betelgeuse and its red coloration have been noted since antiquity; the classical astronomer Ptolemy described its color as ὑπόκιρρος. It was described by a translator of Ulugh Beg's Zij-i Sultani as rubedinem "ruddiness".[56]
Herschel's discovery
[edit]
The variation in Betelgeuse's brightness was first described in 1836 by Sir John Herschel, when he published his observations in Outlines of Astronomy; he noted an increase in activity from 1836–1840, followed by a subsequent reduction. In 1849, he noted a shorter cycle of variability which peaked in 1852. Later observers recorded unusually high maxima with an interval of several years, but only small variations from 1957 to 1967. The records of the American Association of Variable Star Observers (AAVSO) show a maximum apparent magnitude (brightness) of 0.2 in the years 1933 and 1942, with a minimum fainter than magnitude 1.2 in both 1927 and 1941.[28][29] This variability in brightness may explain why Johann Bayer designated the star alpha as it may have rivalled the usually brighter Rigel (beta).[57]
In 1919, Albert Michelson and Francis Pease mounted a 6-metre (20 ft) interferometer on the front of the 2.5 metre (100 inch) telescope at Mount Wilson Observatory. Helped by John Anderson, in December 1920 the trio measured the angular diameter of α Orionis as 0.047"—a figure which resulted in a diameter of 3.84 × 108 km (240 million miles or 2.58 AU) based on the then-current parallax value of 0.018".[58] However, there was known uncertainty owing to limb darkening and measurement errors—a central theme which would be the focus of scientific inquiry for a whole century. Beginning with this first measurement at visible wavelengths, researchers have conducted multiple experiments ranging from the ultraviolet to the far infrared, with each producing different results fueling much debate.
The 1950s and '60s saw several important developments, the two Stratoscope projects and the 1958 publication of Structure and Evolution of the Stars, both the work of Martin Schwarzschild.[59] The book taught a generation of astrophysicists how to use emerging computer technology to create stellar models while the Stratoscope projects, by taking instrumented balloons above the Earth's turbulance, produced some of the finest images of solar granules and sunspots ever seen, thus confirming the existence of convection in the solar atmosphere. Both developments would prove to have a significant impact on our understanding of the structure of red supergiants like Betelgeuse.
Aperture masking
[edit]In the late 1980s and early 1990s, Betelgeuse became a regular target for aperture masking interferometry visible-light and infrared imaging, which revealed a number of bright spots on the star's surface, thought to result from convection.[61][62] These were the first optical and infrared images of the disk of a star other than our Sun, and generally showed one or more bright patches—indicating the location of hotspots in the star's photosphere.[63]
In 1995, the Hubble Space Telescope's Faint Object Camera captured an ultraviolet image of comparable resolution—this was the first conventional-telescope image (or "direct-image" in NASA terminology) of the disk of another star. The image was taken at ultraviolet wavelengths since ground-based instruments cannot produce images in the ultraviolet with the same precision as Hubble. Like earlier images, this ultraviolet image also contained a bright patch, indicating a hotter region of about 2,000 Kelvin, in this case on the southwestern portion of the star's surface.[64] Subsequent utraviolet spectra taken with the GHRS suggested that the hot spot was one of Betelgeuse's poles of rotation. This would give the rotational axis an inclination of about 20° to the direction of Earth, and a position angle from celestial North of about 55°.[65]
Recent studies
[edit]
Recent ground-based measurements of the disk of Betelgeuse in the mid-infrared, at 11.15 μm, gave an angular diameter of 54.7 ± 0.3 milliarcseconds (mas) in November 1999, slightly smaller than the typical visible-light angular diameter. This measurement assumes a uniform disk model and ignores any possible contribution from hotspots (which are less noticeable in the mid-infrared.) Allowing for the limb darkening effect, whereby the intensity of a star's image diminishes near the edge, gives an increase of approximately 1%, to 55.2 ± 0.5 milliarcseconds (mas). It is difficult to define the precise diameter of Betelgeuse as the photosphere has no "edge"—instead the gas making up the photosphere gets gradually thinner with distance from the star.[3]
On June 9, 2009, Nobel Laureate Charles Townes announced that the star has shrunk 15% since 1993 with an increasing rate. He presented evidence that UC Berkeley's Infrared Spatial Interferometer (ISI) atop Mt. Wilson Observatory had observed 15 consecutive years of stellar contraction. The average speed at which the radius of the star has been shrinking during this period is around 1,000 km/hr.[note 4] Despite Betelgeuse's diminished size, Townes and his colleague, Edward Wishnow, pointed out that the star's visible brightness, or magnitude, which is monitored regularly by members of the American Association of Variable Star Observers (AAVSO), had shown no significant dimming over that same time frame. According to the university, Betelgeuse's diameter is about 5.5 AU, and the star's radius has shrunk by a distance equal to half an astronomical unit, or about the orbit of Venus.[7][66] Townes concluded it was unclear whether the shrinkage is part of a periodic process or not; if it is, then the cycle is likely to be decades long.[67]
In July 2009, images released by European Southern Observatory, taken by the ground based Very Large Telescope, gave a more detailed view of the surface of the star.[31] In the picture a plume of gas six times its photospheric diameter is seen extending from the star.[35] This is comparable to the distance between the Sun and Neptune.
A series of spectropolarimetric observations, obtained in 2010 with the Bernard Lyot Telescope at Pic du Midi Observatory, revealed the presence of a weak magnetic field at the surface of Betelgeuse, suggesting that the giant convective motions of supergiant stars are able to trigger the onset of a small-scale dynamo.[68]
Visibility
[edit]- Section Purpose: Explaining the OBSERVABLE aspects of the star
(already exported)
Enigmatic Parallax
[edit]- Sub-Section Purpose: Explaining the enigma of distance
(already exported)
Enigmatic Parallax2
[edit]The uncertainty of Betelgeuse's distance has puzzled astronomers for centuries, and has made reliable estimates difficult for many other stellar parameters such as luminosity. When combined with the angular diameter of the star, one can estimate the radius and its effective temperature; luminosity combined with an understanding of isotopic abundances provides an estimate of the stellar age and mass.[69] In 1920, when the first interferometric studies were performed, the assumed parallax was 0.180 arcseconds. That equates to a distance of 56 pc or roughly 180 ly. Since then, there has been an ongoing inquiry as to the actual distance of this mysterious star with estimated distances as high as 900 light years being proposed.[69]
Before the publication of the Hipparcos Catalogue (1997), there were two respected publications with up-to-date parallax data on Betelgeuse. The first was the Yale University Observatory (1991) with a published parallax of π = 9.8 ± 4.7 milliarcseconds, yielding a distance of roughly 102 pc or 330 ly.[70] The second was the Hipparcos Input Catalogue (1993) with a trigonometric parallax of π = 5 ± 4 mas, a distance of 200 pc or 650 ly—almost twice the Yale estimate.[71] With such uncertainty, researchers were adopting a wide range of distance estimates, a phenomenon which fueled much debate—not only in terms of the star's distance, but also in terms of its many other implications.[69]

The long-awaited results from the Hipparcos mission were finally released in 1997. Instead of resolving the issue, a new parallax figure was published of π = 7.63 ± 1.64 mas, which equated to a distance of 131 pc or roughly 430 ly.[72] Because stars like Betelgeuse vary in brightness, they raise specific problems in quantifying their distance.[73] As a result, the large cosmic error in the Hipparcos solution may be of stellar origin, perhaps related to movements of the photocenter, of order 3.4 mas, in the Hipparcos photometric Hp band.[69][74]
Where a breakthrough in this debate appears to have come is with the advances in radio astronomy. New high spatial resolution, multiwavelength, NRAO Very Large Array (VLA) radio positions of Betelgeuse have produced a more precise estimate, which combined with the recent Hipparcos data furnished a new astrometric solution: π = 5.07 ± 1.10 mas, a tighter error factor yielding a distance of 197 +/- 45 pc or 643 +/- 146 ly.[69]
In 2012, the European Space Agency's (ESA) upcoming Gaia mission will explore the detailed physical properties of each star observed, revealing luminosity, temperature, gravity and composition. Gaia will achieve this by repeatedly measuring the positions of all objects down to magnitude 20, and those brighter than magnitude 15, to an accuracy of 24 microarcseconds—akin to measuring the diameter of a human hair from 1000 km away. On-board detection equipment will ensure that variable stars like Betelgeuse will all be detected and catalogued to this faint limit, thus addressing most of the limitations of the earlier Hipparcos mission. The nearest stars, in fact, will have their distances measured to the extraordinary accuracy of 0.001%. Even stars near the Galactic centre, some 30 000 light-years away, will have their distances measured to within an accuracy of 20%.[75]
Rhythmic dance
[edit]- Sub-Section Purpose: Variability, Why, Specifics.

- Paragraph Purpose: Explain the basic why of variability
Distance is not the only mystery that has bewitched astronomers. Known as a semiregular pulsating variable, researchers have offered different hypotheses to explain α Ori's volatile choreography. Our current understanding of stellar structure suggests that the outer layers of this supergiant gradually expand and then contract causing the surface area (chromosphere) to alternately increase and decrease, the temperature to rise and fall — thus eliciting the measured cadence in the star's brightness between its dimmest magnitude of 1.2, seen as early as 1927, and its brightest of 0.2, seen in 1933 and 1942. A red supergiant like Betelgeuse will pulsate like this because its stellar atmosphere is inherently unstable. As the star contracts, it absorbs more and more of the energy that passes through it, causing the atmosphere to heat up and expand. Conversely, as the star expands, its atmosphere becomes less dense allowing the energy to escape and the atmosphere to cool, thus initiating a new contraction phase.[28] What's been particularly challenging in this regard has been apprehending the irregularity of the star's pulsations and modeling its periodicity, as it appears there are several cycles interlaced.
*Paragraph Purpose: Explain periodicity Next Step: Need to pull together all the different periodicity estimates from different articles.
Let us first recall that Alpha Orionis varies in both visual brightness and radial velocity on time scales of a few weeks ormonths. As shown in classical papers, the short term fluctuations modulate a regular, cyclic variation with a period of nearly six years. Stebbins (1931) monitored the brightness of Betelgeuse photoelectrically more or less continuously, weather and seasons permitting, from 1917 to 1931. He concluded that "there is no law or order in the rapid changes of Betelgeuse" and used a drastic smoothing process to derive a mean cure that was best fitted by a period of 5.40 days, although the period of 5.781 days, which had been obtained by Spencer Jones (1928) from radial-velocity data, represented the brightness data nearly as well. The observations from Stebbins and Sanford indicate a possible sub-period of about 100 days in the brightness variations.[76] It is noteworthy that on at least 3 occasions during the past 60 years, unusually large and relatively rapid decreases in the radial velocity of Alpha Ori were followed by suddendecreases of about half a magnitude in visual brightness. Such events seem to have a tendency to occur just after the time of the minimum in the radial velocity connected with the star's pulsations. As the pulsating star reaches maximum velocity of expansion and begins to decelerate there may be instabilities set up in the atmosphere which trigger mass ejection adn the formation of dust grains. The correlation between the observed angular diamter of Betelgeuse and the opacity of the TiO (Balega et al. 1982) indicates that the scale height of the region in which the Fraunhofer spectrum is formed is on the order of one stellar radius. The extrem widths of the line in the Alpha Ori and other supergians suggest that the levitation of the atmosphere may be caused by nonthermal gas pressure, but no matter what holds up the atmosphere, its coupling to the pulsating star cannot be very tight and there will be a tendency for the atmosphere to continue accelerating and to over shoot the minimum, as may have been observed. A survey of variations in the radial-velocity and visual brightness of the star Betelgeuse (alpha-Orionis) over the last six decades is presented. On the basis of a comparison of the results of several observations, it is suggested that major disturbances in Betelgeuse's atmosphere are likely to occur in the year or two following the minimum in the six-year velocity curve. A coordinated observing program is proposed to take place during and after the next minimum, which is predicted to take place in early 1984. The desirable observations include multicolor photometry (particularly in the infrared), polarization measurements, and spectroscopy.
As discussed in classical papers by Stebbins and Sanford in the 1930s, there are short term variations of roughly 150 to 300 days that modulate a regular cyclic variation with a period of roughly 5.7 years. In 2000, astronomers announced the detection of statistically significant cycles in "longwave" (1900-3200 Å) ultraviolet light radiated by the star at 0.531 years (just over six months) and 3.46 years Rinehart et al., 2000).[77]

1. Core
2. Radiative zone
3. Convective zone
4. Photosphere
5. Chromosphere
6. Corona
7. Sunspot
8. Granules
9. Prominence
- Paragraph Purpose: Explain complexity/convection
In fact, the supergiant consistently displays irregular photometric, polarimetric and spectroscopic variations, which points to the occurrence of complex activity on the star's surface and in its extended atmosphere.[61] In marked contrast to most giant stars that are typically long-period variables with reasonably regular periods, red giants and supergiants like Betelgeuse and Antares are generally semi-regular or irregular with pulsating characteristics. In a landmark paper published in 1975, Martin Schwarzschild attributed these brightess fluctuations to the changing granulation pattern formed by a few giant convection cells covering the surface of these stars.[33][78] For the Sun, these convection cells, otherwise known as solar granules, represent the foremost mode of heat transfer — hence those convective elements which dominate the brightness variations in the solar photosphere.[33] The typical diameter for a solar granule is about 2,000 km (yielding a surface area roughly the size of India), with an average depth of 700 km. With a surface of roughly 6 trillion square kilometers, there are about 2 million of such granules lying on the Sun's photosphere. Beneath these granules, it is conjectured that there are 5-10 thousand supergranules, the average diameter of which is 30,000 km with a depth of about 10,000 km.[79] By contrast, stars like Betelgeuse, Schwardschild argues, may have only a dozen monster granules with diameters of 180,000,000 km or more dominating the surface of the star with depths of about 60,000,000 kilometers, which, because of the very low temperatures and extremely low density found in red giant envelopes, result in convective inefficiency. Consequently, if only one-third of these convective cells are visible to us at any one time, the time variations in their observable light may well be reflected in the brightness variations of the integrated light of the star.[33]
- Paragraph Purpose: Schwarzschild's impact
Schwarzschild's hypothesis of gigantic convection cells dominating the surface of red giants and supergiants seems to have stuck with the astronomical community. In 1995, the Hubble Space Telescope captured the first direct picture of the supergiant's surface. The image revealed an extended chromosphere of roughly twice the star's angular diameter with a mysterious hot spot located in the southwest quadrant of the disk that dominated the total ultraviolet flux. The hot spot appeared to be hotter than the surrounding chromosphere by at least 2,000K. "Such a major single feature is distinctly different from scattered smaller regions of activity typically found on the Sun", authors Andrea Dupree and Ronald Gilliland reported, "although the strong ultraviolet flux enhancement is characteristic of stellar magnetic activity. This inhomogeneity may be caused by a large scale convection cell or result from global pulsations and shock structures that heat the chromosphere."[80][28] Two years later in 1997, astronomers observed intricate assymetries in the brightness distribution of the star, revealing at least three bright spots on the stellar disk.[81] And then in the year 2000, another team of astronomers led by Alex Lobel of the Harvard–Smithsonian Center for Astrophysics (CfA) noted that Betelgeuse exhibits raging storms of hot and cold gas in its turbulent atmosphere, a potential cause being asymmetric pulsations in the star's chromosphere. The team surmised that huge areas of the star's photosphere will vigorously bulge out in different directions at times, ejecting long plumes of warm gas into the cold dust envelope. Another explanation that was also given was the occurrence of shockwaves caused by warm gas traversing cooler regions of the star.[82][77] The team investigated the atmosphere of Betelgeuse, over a period of five years between 1998 and 2003 with the STIS instrument aboard Hubble. They found that the bubbling action of the chromosphere tosses gas out one side of the star, while it falls inward at the other side, similar to the slow-motion churning of a lava lamp.
*Paragraph Purpose: Latest findings that explain variability Next Step: Read Dupree and other recent articles; integrate

A Harvard-Smithsonian Center for Astrophysics Press Release announcing the first direct image of a star (Betelgeuse), suggests that this event holds new implications for stellar research. Researchers said that this new picture of Betelgeuse suggests that a totally new physical phenomenon may be affecting the atmospheres of some stars. "What we see on Betelgeuse is completely different from what occurs on the surface of the Sun," said Dupree, a senior scientist at the CfA. "Instead of lots of little sunspots, we find an enormous bright area more than 2,000 K degrees hotter than the surrounding surface of the star." Until follow-up observations of Betelgeuse are completed, however, astronomers won't know the mystery spot's true nature--or how it formed.[28]
Dupree explains that the spot might change position over time. Dupree and colleagues using the International Ultraviolet Explorer found a 420-day period, during which the star oscillates, or "rings" like a bell. The oscillations, thought to be caused by turbulence below the surface of the star, might cause changes in the bright spot. Future oscillations might spur other bright spots on different regions of the star's surface, causing it to wink on and off like blinking lights on a Christmas tree, said Dupree. Alternatively, the spot might move systematically across the star's surface, which would imply that the star has magnetic fields strong enough to hold the bright spot's hot gas in position. Either scenario would lead astronomers to re-think completely current ideas of how stars evolve. "We hope this work will also pave the way for a generation of space interferometers," said Dupree. Such instruments would greatly improve the resolution of structures on stellar surfaces.[28]
The major question this hot spot raised was whether it was linked to oscillations previously detected in the giant star, or whether it somehow moves systematically across the star's surface under the grip of powerful magnetic fields.[28]
- Paragraph Purpose: Summarize section and explain major Starbox distinctions Next Step: Better summary of previous material. This section may be better at the beginning of the Properties section.
As a variable star, Betelgeuse has the sub-classification "SRC": supergiants with amplitudes of about 1 mag and periods of light variation from 30 days to several thousand days. If we assume a distance of 197 pc (± 640 ly), the peak absolute magnitude of Betelgeuse would be -6.27, while its minimum would be -5.27, and its mean -6.05.
Rhythmic Source Data
[edit]- Townes 2009:In 2006, the ISI detected a major hot spot on α Ori, producing about 10% of the total flux at 11.15 μm, and resulting in some asymmetry in the radiation intensity (Tatebe et al. 2007). This discovery is consistent with theoretical predictions by Schwarzschild (1975).
- SolStation: In 1995, Betelgeuse became the target of the Hubble telescope's first direct image of a star which revealed an enormous hot spot in its atmosphere. Instead of lots of little sunspots as on most main sequence stars, astronomers found an enormous bright area more than 2,000 °K hotter than the surrounding surface of the star. Turbulence below the star's surface may also have been driving a 420-day oscillation (whereby the star "rang" like a bell) and may cause changes in the observed hot spot (more from STScI press release, Astronomy Picture of the Day, and Gilliland and Dupree, 1996).[77]
- Young 2000 We report contemporaneous multi-wavelength interferometric imaging of the red supergiant star Betelgeuse (α Orionis), using the Cambridge Optical Aperture Synthesis Telescope (COAST) and the William Herschel Telescope (WHT), at wavelengths of 700, 905 and 1290 nm. We find a strong variation in the apparent symmetry of the stellar brightness distribution as a function of wavelength. At 700 nm the star is highly asymmetric, and can be modelled as the superposition of three bright spots on a strongly limb-darkened disc. However, at 905 nm only a single low-contrast feature is visible and at 1290 nm the star presents a featureless symmetric disc. The change in spot contrast with wavelength is consistent with a model in which the bright spots represent unobscured areas of elevated temperature, owing perhaps to convection, on a stellar disc that itself has a different appearance, i.e. geometrical extent and limb-darkening profile, at different wavelengths. The featureless centre-to-limb brightness profile seen at 1290 nm is consistent with this model and suggests that future interferometric monitoring of the star to quantify the size changes associated with radial velocity variations should be performed at similar wavelengths in the near-infrared.
Angular anomalies
[edit]- Sub-Section Purpose: Stellar Diameter — Why so many estimates?
A third challenge that has confronted astronomers has been measuring this behemoth's angular diameter. As noted earlier, Betelgeuse became the first star to have its diameter measured on December 13, 1920 by means of a 20-foot beam interferometer.[58] Though interferometry was still very much in its infancy, the experiment proved to be a success and the angular diameter was found to average .047 arcseconds. What was noteworthy were the astronomers' insights on limb darkening. In addition to a measurement error of 10%, Michelson and Pease estimated the actual size of the star to be about 17% larger because of the diminishing intensity of light around the edges—hence an angular diameter as large as .055".[58][13] Since that time, there have been many other studies conducted with angles ranging anywhere from .042 to .069 arcseconds.[3][83][84] If we simply take that data and combine it with historical distance estimates of 180 to 815 ly, the projected diameter of the stellar disk could be anywhere from 2.4 to 17.8 AU, hence radii of 1.2 to 8.9 AU respectively.[note 2] That's a wide margin—hence one of the reasons Betelgeuse has been such a mystery. Using the Solar System as a yardstick, the orbit of Mars is approximately 1.5 AU, Ceres in the asteroid belt 2.7 AU, Jupiter 5.5 AU—hence a photosphere which, depending on Betelgeuse's actual distance from Earth, could well extend beyond the orbit of Jupiter but not quite as far as Saturn at 9.5 AU.

The precise diameter has been hard to define for several reasons:
- The rhythmic expansion and contraction of the photosphere means the diameter is never constant;
- There is no definable "edge" to the star as limb darkening causes the optical emissions to vary in color and decrease the farther one extends out from the center;
- Betelgeuse is surrounded by a circumstellar envelope composed of matter being ejected from the star—matter which both absorbs and emits light—making it difficult to define the edge of the photosphere;
- Measurements can be taken at varying wavelengths within the electromagnetic spectrum, with each wavelength revealing something different. Observed angular diameters are significantly larger in the visible, decrease to a minimum in the near-infrared, and then increase again in the mid-infrared.[6][85] The difference in reported diameters can be as much as 30–35%, yet because each wavelength measures something different, comparing one finding with another can be difficult.[7]
- Atmospheric twinkling limits the resolution obtainable from ground-based telescopes since turbulence in the Earth's atmosphere degrades the angular resolution.[61]
To overcome these challenges, researchers have employed a number of solutions. Astronomical interferometry was first imagined by Hippolyte Fizeau in 1868.[86] He proposed the observation of stars through two apertures to obtain interferences that would provide information on the spatial intensity distribution of the source. Since then, the science of interferometry has evolved considerably where multiple-aperture interferometers are now used consisting of a large number of images superimposed on each other. These "speckled" images are then synthesized using Fourier analysis—a method which has been used for a wide array of astronomical objects including the study of binary stars, quasars, asteroids and galactic nuclei.[87] Space observatories like Hipparcos, Hubble and Spitzer have produced significant breakthroughs and recently another instrument, the Astronomical Multi-BEam Recombiner (AMBER), is yielding new insights. As part of the VLTI, AMBER is capable of combining the beams of three telescopes simultaneously, allowing researchers to achieve milliarcsecond spatial resolution. Also by combining three baselines instead of two, which customary with conventional interferometry, AMBER enables astronomers to compute the closure phase—an important element in astronomical imaging.[85][9]
The current debate centers around which wavelength—the visible, near-infrared (NIR) or mid-infrared (MIR)—produces the most accurate angular measurement.[note 2] The solution that has been most widely adopted, it appears, is the one performed with the ISI in the mid-infrared by astronomers from the Space Sciences Laboratory at U.C. Berkeley. In the epoch year 2000, the group, under the leadership of John Weiner, published a paper showing Betelgeuse as having a uniform disk of 54.7 ± 0.3 mas.[3] The paper also included a theoretical allowance for limb darkening yielding a diameter of 55.2 ± 0.5 mas—a figure which equates to a radius of roughly 5.5 AU (1,180 times solar), assuming a distance of 197.0 ± 45 pc.[note 3] Nevertheless, given the angular error factor of ± 0.5 mas combined with a parallax error of ± 45 pc found in Harper's numbers, the photosphere's radius could actually be as small as 4.2 AU or as large as 6.9 AU.
Crossing the Atlantic, another team of astronomers led by Guy Perrin of the Observatoire de Paris produced a document in 2004 arguing that the near-infrared figure of 43:33 ± 0:04 mas was a more accurate photospheric measurement. "A consistent scenario to explain the observations of this star from the visible to the mid-infrared can be set-up", Perrin reports. "The star is seen through a thick, warm extended atmosphere that scatters light at short wavelengths thus slighty increasing its diameter. The scatter becomes negligible above 1.3 μm. The upper atmosphere being almost transparent in K and L—the diameter is minimum at these wavelengths where the classical photosphere can be directly seen. In the mid-infrared, the thermal emission of the warm atmosphere increases the apparent diameter of the star." It's a compelling argument but one which has yet to receive widespread support among astronomers.[7]
More recent studies done in the near-infrared with the IOTA and VLTI have brought strong support to Perrin's analysis yielding diameters that range from 42.57 to 44.28 mas with impressively tight error factors of no more than 0.04 mas.[9][10] Of pivotal importance in this discussion, however, is a second paper published by the Berkeley team in 2009, this time led by Charles Townes, reporting that the radius of Betelgeuse had actually shrunk from 1993 to 2009 by 15%, with the 2008 angular measurement equal to 47.0 mas, not too far from Perrin's estimate.[13][14] Unlike most papers heretofore published, this seminal paper represented a systematic study of the star over a 15-year horizon at one specific wavelength. Earlier studies have typically lasted one to two years by comparison and have explored multiple wavelengths, often yielding vastly different results. The diminution in angular separation equates to a range of values between 56.0 ± 0.1 mas seen in 1993 to 47.0 ± 0.1 mas seen in 2008—a contraction of almost 0.9 AU.[note 4] What is not fully known is whether this observation is evidence of a rhythmic expansion and contraction of the star as astronomers have theorized, and if so, what the periodic cycle might be, although Townes suggests that if a cycle does exist, it is likely a few decades long.[13] Consequently, until a full cycle of data has been gathered, we will not know whether the 1993 figure of 56.0 mas represents the maximum extension of the star or its mean, or whether the 2008 figure of 47.0 in fact represents a minimum. It will likely take another 15 years or longer (2025 C.E.) before we know with any certainty, meaning that the Jovian orbit of 5.5 AU will likely serve as the star's "average" diameter for some time.[5]
Once considered as having the largest angular diameter of any star in the sky after the Sun, in 1997 Betelgeuse lost that distinction when a group of astronomers measured R Doradus with a diameter of 57.0 ± 0.5 mas. Betelgeuse is now considered to be in third place, although R Doradus, being much closer to Earth at about 200 ly, has an actual diameter roughly one-third that of Betelgeuse.[17] _____________END OF SECTION______________________________________________________________
Original Language
[edit]Because of the size and proximity of this star it has the third largest angular diameter as viewed from Earth, being smaller than only the Sun and R Doradus. The angular size of Betelgeuse was one of the first to be measured with an astronomical interferometer and the apparent diameter was found to be variable. Between 1993 and 2009, the star's diameter has contracted by over 15 percent.[88] The precise diameter is hard to define since optical emissions decrease very gradually with radius from the center of Betelgeuse and the color of these emissions also vary with radius.
If we assume a compromise distance of 570 light years, the star's diameter would be about 950 to 1,000 times that of the Sun. If the Sun were the size of a beach ball, then Betelgeuse would be as large as a professional sports stadium. Although only 20 times more massive than the Sun,[27] this star could be hundreds of millions times greater in volume.
Angular Source Data
[edit]- ESO/AMBER 2007: AMBER is part of the VLT Interferometer (VLTI) and completes the planned set of first-generation instruments for this facility. It continues the success story of the interferometric mode of the VLT, following the unique initial scientific results obtained by the VINCI and MIDI instruments, the installation of the four MACAO adaptive optics systems and the recent arrival of the last of the four 1.8-m Auxiliary Telescopes at Paranal. The principle of the interferometric technique is to combine the light collected by two or more telescopes. The greater the distance between the telescopes, the more details one can detect. For the VLTI, this distance can be up to 200 metres, providing observers with milli-arcsecond spatial resolution. With such a high spatial resolution, one would be able to distinguish between the headlights of a car located on the Moon. In addition, AMBER also provides astronomers with spectroscopic measurements, allowing the structure and the physics of the source to be constrained by comparing the measures at different wavelengths. AMBER combines the light beams from three telescopes - this is a world first for large telescopes such as the VLT. The ability to combine three beams, rather than just two as in a conventional interferometer, provides a substantial increase in the efficiency of observations, permitting astronomers to obtain three baselines simultaneously instead of one. The combination of these three baselines also permits the computation of the so-called closure phase, an important mathematical quantity that can be used in imaging applications.
- Michelson 1920: Assuming that the effective wavelength for α Orionis is λ5750, its angular diameter from the formula a=1.22 λ/β proves to be 0".047; and with a parallax of 0".018 its linear diameter turns out to be 240 X 106 miles, or slightly less than that of the orbit of Mars. This value corresponds to a uniformly illuminated disk, while for one darkened at the limb, this result, as mentioned above, would be increased by 17 per cent. The uncertainty of the measurement of the angular diameter is about 10 per cent.[58]
- Bonneau 1973: Interferometric estimates of Betelgeuse's diameter ranging between 52:0 ± 5.0 mas and 69:0 ± 5.0 mas. Bonneau's conclusion: Few models of cool stars have been published (i.e. 1921-1970), probably because of the extreme complexity of transfer phenomena in extended stellar atmospheres containing not only atoms and molecules but also perhaps solid grains assembled in shell or dish structures. Clearly, the limb-darkening effects to be expected from such objects depend greatly on the relative contribution of absorption and scattering phenomena, such as Rayleigh, Mie, or resonances cattering, in their atmospheres. However, spectroscopy has not given much information on the scattering properties of stellar atmospheres.[83]
- Balega 1982: The averaged value of diameter measurements in Table 1 is 57 ± 8 m; the weighted average is not different: 58m". The mean error bars is 3 m". Our mean value of the diameter of Betelgeuse is relatively large compared to previous measurements, down to 34 m" by Pease (Sanford, 1933); but diameters as large as 69 m" have been reported (Bonneau and Labeyrie, 1973).[84]
- Bedding 1997: We find the angular diameter of R Doradus to be 57+/-5mas, exceeding that of Betelgeuse and implying that R Dor is larger in apparent size than every star except the Sun. R Dor is shown to be closely related to the Mira variables. We estimate an effective temperature of 2740+/-190K, a distance of 61+/-7pc, a luminosity of 6500+/-1400 Lsolar and a radius of 370+/-50 Rsolar. The characteristics of R Dor are consistent with it being near the edge of a Mira instability strip. We detect non-zero closure phases from R Dor, indicating an asymmetric brightness distribution.
- Weiner 2000: Based on fitting the visibility data to uniform disk models, the diameter of α Orionis is 54.7 ± 0.3 mas and that of ο Ceti at phase 0.90 is 47.8 ± 0.5 mas. These diameters are the most precise ever measured in the mid-infrared, due in part to the addition of a 56 m baseline to the ISI, which provided sufficient resolution to observe the first zero in the visibility function of the stellar disks. Moreover, the effects of limb darkening and stellar hot spots are small at these wavelengths. Theoretically estimated limb-darkening effects indicate the actual diameters are approximately 1%+/-0.5% larger than the uniform disk approximation, or 55.2 ± 0.5 and 48.2 ± 0.6 mas for α Orionis and ο Ceti, respectively.[3]
- Perrin 2004: Because of their large luminosity supergiant stars have extended atmospheres, with resultant low surface gravity and large pressure scale height. The cool supergiants are usually slightly variable in brightness, color, polarization, and radial velocity, suggestive of significant dynamic and radiative activity and inhomogeneities. However, the variability is small enough that static model atmospheres are normally considered adequate for abundance studies. Owing to the very extended atmospheres, and in some cases circumstellar shells, it is difficult to determine even some very basic parameters for these stars. Here, we address the question of the effective temperatures. The near infrared wavelength regime offers several advantages for the study of cool supergiants - it is less obscured by scattering and line blanketing (problems in the visible) and by dust (detected in the mid-infrared).
- Perrin 2004: The observed angular diameters are significantly larger in the visible, decrease to a minimum in the near-infrared, and then increase again in the mid-infrared. Some surface structure, similar to large spots, has been seen at several wavelengths in the red (Tuthill et al. 1997; Young et al. 2000). These spots have not been seen at other nearby wavelengths.[6]
- Perrin 2004: The K and L band diameters found by our group are identical to within the errors of differential wavelength calibration between TISIS and FLUOR. We have used the L band measurement of March 2000 reported in Chagnon et al. (2002). The diameter of mas is slightly smaller than the K band diameter but we think this is due to the poorer L band calibration and to the much larger uncertainty on effective wwavelength. This situation of very close diameters in K and L is dramatically different from what we have found for Mira type stars, and can be compared to what could be expected for non pulsating giant stars. Yet, in the case of Betelgeuse, measurements at 11.15 m by Weiner et al. (2000) show a visibility curve very similar to that expected of a photosphere with a diameter 25% larger than the diameter we find at two shorter wavelengths. Two different explanations may be invoked. The first one would be that the limb-darkening might be very strong at shorter wavelengths mimicking a much smaller apparent diameter. This is the explanation of Weiner et al. (2000). However, this is not compatible with the value of limb-darkening we find in the K band and with the shape of the visibility curve which should be very much altered by such a huge effect. Another possibility may be suggested from the study of the atmosphere of Mira stars. We have observed the brightest Mira stars both in the L band (Mennesson et al. 2002) and in the molecular and continuum bands in K (Perrin et al. 2004a). We have shown that the visibility data could be understood in all bands if a warm molecular layer were added around the star. Because of the huge amount of gas around Mira stars (perhaps elevated by dynamical effects associated with pulsation), the apparent diameters in the K and L bands are very different and much bigger in L due to the lower brightnesss contrast between the molecules and the photosphere of the star. The same feature can be tentatively invoked for Betelgeuse to explain the different diameters from K and L to 11.15 m. Narrow band near IR measurements are not yet available for Betelgeuse, but we acquired such measurements on another supergiant, Cep, which is similar in major respects to Orionis, including evidence for substantial mass loss. Features similar to Mira stars were detected in the visibility measurements of Cep (Perrin et al. 2004b). This may also apply to Betelgeuse. As a consequence, we have chosen to portray the model as a molecular layer in the following, which appears consistent with other evidence. An interpretation in terms of dust would also be possible.[6]
- Hernandez 2009: The apparent size and greater luminosity of Red Super Giant stars (RSGs) make them ideal targets for astrometry experiments. Since the observation of Betelgeuse by Michelson & Pease (1921), several other works have been carried out improving the precision on the measurement of the diameter of the star (e.g., Weiner et al. 2000; Young et al. 2000). We present preliminary results of the observations of the super giant star Betelgeuse carried out with the AMBER (Astronomical MultiBEam Recombiner) instrument. AMBER is one of the first generation instruments of the VLTI able to combine the beams of two or three telescopes simultaneously, and brings spectral dispersion. The a priori knowledge of the angular size of Betelgeuse, gives us a well established reference to indirectly probe the stability of AMBER. Furthermore, we demonstrate the AMBER ability to measure low contrast visibilities under high flux conditions. The calibrated visibilities of Betelgeuse are plotted with the corresponding uniform disk model in Figure 2, and the diameter is found to be: UD = 42:57 ± 0:02 mas We verify the consistency of our result by comparing it with those previous published in the literature. The observations of Betelgeuse carried out by Perrin et al. (2004) with the IOTA interferometer are the most relevant to our study. They report a diameter of 43:33 ± 0:04 mas.[9]
- Kervella 2009: Context: Betelgeuse is one the largest stars in the sky in terms of angular diameter. Structures on the stellar photosphere have been detected in the visible and near-infrared as well as a compact molecular environment called the MOLsphere. Mid-infrared observations have revealed the nature of some of the molecules in the MOLsphere, some being the precursor of dust. Aims: Betelgeuse is an excellent candidate to understand the process of mass loss in red supergiants. Using diffraction-limited adaptive optics (AO) in the near-infrared, we probe the photosphere and close environment of Betelgeuse to study the wavelength dependence of its extension, and to search for asymmetries. Methods: We obtained AO images with the VLT/NACO instrument, taking advantage of the “cube” mode of the CONICA camera to record separately a large number of short-exposure frames. This allowed us to adopt a “lucky imaging” approach for the data reduction, and obtain diffraction-limited images over the spectral range 1.04-2.17~μm in 10 narrow-band filters. Results: In all filters, the photosphere of Betelgeuse appears partly resolved. We identify an asymmetric envelope around the star, with in particular a relatively bright “plume” extending in the southwestern quadrant up to a radius of approximately six times the photosphere. The CN molecule provides an excellent match to the 1.09 μm bandhead in absorption in front of the stellar photosphere, but the emission spectrum of the plume is more difficult to interpret. Conclusions: Our AO images show that the envelope surrounding Betelgeuse has a complex and irregular structure. We propose that the southwestern plume is linked either to the presence of a convective hot spot on the photosphere, or to the rotation of the star.
- SolStation: Various measures, particularly those made at higher energy wavelengths where the star's outer gases are particularly opaque, suggest an enormous size (more discussion). In red light, Betelgeuse has an angular diameter of 0.054 arcseconds at 720 nm (Wilson et al., 1992), so that it would extend to 3.6 AUs into the Main Asteroid Belt within the Solar System (for a diameter of 7.2 AUs in red light). In more energetic ultraviolet light, its radius has been measured at over eight AUs out (for a diameter of 16 AUs), or more than twice that at optical wavelengths. Finally, the large radio photospheres of red giant and supergiant stars give rise to detectable thermal radio emission, so that in radio wavelengths, the star's has been found to extend beyond Saturn's orbit (more discussion). Further ultraviolet and far ultraviolet images of the star revealed a chromospheric diameter as large as 260 to 270 milli-arcseconds, or 34 to 35 AUs -- between the orbits of Neptune and Pluto (Timothy et al., 2002; and Uitenbroek et al., 1998).[77]
Properties
[edit]- Section Purpose: Explore DETAILS section of Starbox
Betelgeuse is not only one of the most eminent stars in the heavens, but also one of the most luminous. In the SIMBAD astronomical database the star's spectral class is listed as M2Iab. The "ab" suffix is derived from the Yerkes spectral classification system signifying that Betelgeuse is an intermediate luminous supergiant, less luminous than other supergiants like Deneb. However given some of the recent findings, it may be that this classification is outdated, as Betelgeuse, it appears, is actually much brighter than Deneb and other stars in its class.
If we assume an average radius of 5.5 AU and a distance of 197 pc, Betelgeuse has a luminosity in excess of 180,000 Suns at maximum. When the star contracts as it has since 1993, its luminosity diminishes to about 130,000 Suns. Either way, that amount of electromagnetic energy certainly dwarfs Deneb's output of about 50,000 Suns.[note 1] However, with most of the star's radiant energy occurring in the infrared and huge amounts of it being absorbed by circumstellar matter in the star's outer shell, we simply don't experience the star's total luminosity.
Betelgeuse is a cool star, typical of red supergiants, with a surface temperature of about 3,500 degrees Kelvin. It's also a slow rotator, with the most recent velocity recorded at 5 km/s. At 5.5 AU, it takes the star roughly 32.3 years to turn on its axis — extremely slow when you compare it to a fast rotator like Pleione in the Pleiades star cluster which turns on its axis once every 11.8 hours.
Source Data
- Intro Section Purpose: Discuss extraneous Starbox details that have no sub-section
- Temperature. The surface temperature is that of a typical M-type red supergiant, about 3500 degrees Kelvin.
- Luminosity.
- Metalicity.
- Rotation.
- Classification. In the SIMBAD astronomical database the star's spectral class is listed as M2Iab. The "ab" suffix is derived from the Yerkes spectral classification system signifying that Betelgeuse is an intermediate luminous supergiant, less luminous than other supergiants like Deneb.
- AAVSO: Betelgeuse is not only among the largest, but also one of the most luminous stars of its class. At a distance of roughly 425 light-years, it is the seventh brightest star in the northern hemisphere. Its diameter, based on the new distances recently reported by the European Space Agency satellite HIPPARCOS, is about 1500 times the diameter of our Sun (Press Release, 1996). Betelgeuse has a luminosity of about 14,000 Suns at maximum and 7600 Suns at minimum. The peak absolute magnitude of Betelgeuse is about -5.6. The surface temperature is that of a typical M-type red supergiant, about 3100 degrees Kelvin. Only about 13% of the radiant energy is emitted in the form of visible light, so if our eyes were sensitive to radiation at all wavelengths, Betelgeuse would appear as the brightest star in the sky.[29][28]
Cryptic pathways
[edit]- Intro Section Purpose: Age, Origin, Evolution
The kinematics of Betelgeuse are intriguing yet not easily explained. The age of a Type M supergiant with an initial mass of 20 times solar is roughly 10 million years.[89][69] Given its current space motion, a projection back in time would take Betelgeuse around 290 parsecs farther from the galactic plane where there is no star formation region — an implausible scenario. Although the space motion for the 25 Ori group has yet to be measured, α Ori’s projected path does not appear to intersect with it either. Also, formation close to the far younger Orion Nebula Cluster (ONC, also known as Ori OB1d) is doubtful. VLBA astrometry yields a distance to the ONC between 389 and 414 parsecs. Consequently, it is likely that Betelgeuse has not always had its current motion through space and has changed course at one time or another.[69]
The most likely star-formation scenario for Betelgeuse is that it's a runaway star from the Orion OB1 Association. Originally a member of a high-mass multiple system within Ori OB1a, which includes the late type O and B stars in Orion's belt — Alnitak, Alnilam and Mintaka — Betelgeuse was probably formed about 10-12 million years ago from the molecular clouds observed in Orion but has evolved rapidly due to its unusually high mass.[69]
Like many of the stars of Orion where massive young stars with over 10 times the Sun's mass can be found in abundance, Betelgeuse will use its fuel quickly and not live very long. On the Hertzsprung-Russell diagram, Betelgeuse has moved off the main sequence and has swelled and cooled to become the red supergiant that it known as. Although this titanic star may have only been in existence for ten million years, unlike its OB cousins born about the same time, Betelgeuse has probably exhausted the hydrogen in its core causing it to contract under the force of gravity into a hotter and denser state. As a result, it has begun to fuse helium into carbon and oxygen producing enough radiation to unfurl its outer envelopes of hydrogen and helium. Its extreme luminosity is being generated by a mass so large that the star will eventually fuse higher elements through neon, magnesium, sodium, and silicon all the way to iron, at which point it will likely collapse and explode as a supernova [27][77]
Cryptic Source Data
[edit]- SolStation: Massive stars use their fuel quickly and do not live very long. Although Betelgeuse may only be around six million years old, core hydrogen for thermonuclear fusion has already run out at its core, so that gravity has contracted the core into a hotter and denser state to fuse helium into carbon and oxygen and produce enough radiation to swell out its outer envelopes of hydrogen and helium. The star is relatively rich in nitrogen compared to a less evolved star like Sol (Lambert et al., 1984 ). Its extreme luminosity is probably being generated by a mass so large (e.g., 15 to 17 Solar-masses), so that eventually the star will fuse higher elements all the way to iron and collapse and explode as a supernova (James Kaler, 2002). If Betelgeuse actually has only 12 to 14 Solar-masses, however, it may become one of the exceedingly rare neon-oxygen white dwarfs (rather than the more common ones made mostly of carbon and oxygen nuclei).[77]
- Harper The kinematics of Betelgeuse are intriguing and not easily explained. The space motion is [U', V' , W'] = [−12.7, +0.5, +28.2 km s−1] for a distance of 197 pc. The current galactic coordinates relative to the Sun are [X, Y, Z] = [−182,−66,−31 pc] and therefore projection of Betelgeuse’s space motion back in time only takes the star farther from the galactic plane. The age of an early M supergiant with an initial mass of 20 times solar is roughly 10 million years. (Meynet and Maeder 2003) and Betelgeuse would have moved ∼290 pc farther from the galactic plane over that time interval. Projecting the star’s space motion back in time for 10 Myr does not place it close to any likely star formation region for any adopted distance between 150 and 300 pc. One possible explanation is that Betelgeuse has not followed its current space motion for all its life. If the space motion is projected backwards in time, 2.5 Myr ago Betelgeuse passed through the extensive Ori OB1a star-formation region. Brice˜neno et al. (2005) find an age of 7–11 Myr for Ori OB1a depending on the evolutionary tracks used (7.4 Myr results from the Baraffe et al. (1998) tracks, while 10.7 Myr results from those of Siess et al. (2000)). One of the clearest subassociations within Ori OB1a is the 25 Ori group which has an age of 10 Myr and a distance of 330 pc (Brice˜no et al. 2007) and [X, Y, Z] = [−292,−112,−104 pc]. The space motion of the ONC is approximately [U', V', W'] = [−11.0,−2.4, +1.1 km s−1] (see Sandstrom et al. 2007) and its location is [X, Y, Z] = [−322,−178, −129 pc]. While the ONC lies more than 100 pc from the galactic plane, its motion orthogonal to the galactic plane is very small unlike the motion of Betelgeuse. Figure 4 shows a representation of Betelgeuse’s position as a function of age in comparison with the young ONC and older Ori OB1 association.[69]
Ethereal Cubed
[edit]- Sub-Section Purpose: Mass, Size, Density

As an early M-type supergiant, Betelgeuse is one of the largest and most luminous stars of its class. A radius of 5.5 AU is roughly 1,180 times the radius of the Sun — a sphere so huge that it could contain over 2 quadrillion Earths (2.15 × 1015) or more than 1.6 billion (1.65 × 109) Suns. That's the equivalent of Betelgeuse being a giant football coliseum like Wembley Stadium in London with the Earth a tiny pearl, 1 millimeter in diameter, orbiting a Sun the size of a mango.[note 5] Of particular interest in this respect is the impact of a 15% reduction in the star's radius as reported. That equates to a shortening of the star's radius from about 5.5 AU to 4.6 AU, and a diminution in the star's photospheric volume of approximately 41% or 680 million Suns.[note 6]

Not only is the photosphere enormous, but the star is surrounded by a circumstellar gaseous envelope that extends well over a trillion kilometers from the star. As a result, it takes over two months for light to escape its own shell. In the outer reaches of the photosphere, the density is extremely low. In volume, Betelgeuse exceeds the Sun by a factor of about 1.6 billion Suns. Yet the actual mass of the star is no more than 18-19 solar masses, since the star is estimated to have lost 1-2 solar masses since its birth.[27] Consequently, the average density of this stellar mystery is less than one-sextillionth (1.116 × 10−23) the density of our Sun. If we compare such star matter to the density of ordinary air at sea level, the ratio is roughly 1.286 × 10−5 — a density so tenuous, one would have to get above the noctilucent clouds in the Earth's mesosphere to experience it.[note 7] Such star matter is so ethereal that Betelgeuse has often been called a "red-hot vacuum".[29][28]
Ethereal Source Data
[edit]- AAVSO: The star is one of the largest known; spectroscopic studies show that the diameter of the star may vary by about 60% during the whole cycle, a difference considerably larger than the radius of the Earth's orbit![28]
- SolStation: Betelgeuse is a red supergiant star of spectral and luminosity type M1-2 Ib. The star may have between 12 and 17 times Sol's mass (ESA), over 650 times Sol's diameter, and around 40,000 to 100,000 times its bolometric luminosity.[77]
- Goldberg: Most red giant and supergiant stars are irregular variables and since they also display high rates of mass loss, it might be conjectured that the mass loss is related to the variability.[76][33] suggested that large scale convection might somehow trigger ejection of gas clouds. The visual light of red giants shows linear polarization, which varies on the same time scale as the brightness, and suggests a high degree of asymmetry in the stellar atmosphere.[76]
- SolStation: In results presented on June 9, 2009 at the 214th Meeting of the American Astronomical Society, astronomers (including Charles H. Townes, Edward H. Wishnow, Dale D.S. Hale, and B. Walp) reported that Betelgeuse appears to have decreased its diameter by more than 15 percent since 1993 (UC Berkeley press release; Townes et al., 2009; and Rachel Courtland, New Scientist, June 10, 2009). Past observations have indicated that, partially because of roiling convection cells beneath its surface, the star's surface has been observed to "wobble in and out" along with two pulsations, one restarting annually while the other cycles over six years. Based on long-term monitoring at 11.15 micrometers using the Infrared Spatial Interferometer at Mount Wilson Observatory, however, the star's diameter appears to have progressively shrunk from 11.2 to 9.6 AUs; as the star's radius is now just under about five times Earth's orbit distance, having shrunk by a distance equal to the orbit of Venus. It is still unclear whether the star is experiencing a long-term oscillation in its size, undergoing initial contractions towards a collapse or a blow off of material related to its impending death as a red supergiant via a supernova, or simply rotating to present a different side of its bumpy surface (and so appear to change in size). Although the star appears spherically symmetrical at present, Townes and former graduate student Ken Tatebe had observed a bright spot on the surface of Betelgeuse in recent years.[77]
Circumstellar Dynamics
[edit]- Sub-Section Purpose: 1) Mass Loss, 2) Envelope Structure
In the late phase of stellar evolution, massive stars like Betelgeuse exhibit high rates of mass loss, possibly as much as 1M☉ every 10,000 years, resulting in a complex circumstellar environment that is constantly in flux.[31] All stars exhibit mass loss. Rates vary from about 10−14 to 10−4 yr −1 depending on spectral type, luminosity class, rotation rate, companion proximity, and evolutionary stage.[32] Exactly how this mass loss occurs, however, has been a mystery confronting astronomers for decades. When Schwarzschild first proposed his theory of monster convection cells, he argued it was the likely cause in red supergiants. Prior attempts to explain mass loss in terms of solar wind theory had proven unsuccessful as they led to a contradiction with observations involving circumstellar shells.[33] Other theories that have been advanced include magnetic activity, global pulsations and shock structures as well as stellar rotation.[34]

As a result of work done by Pierre Kervella and his team at the Paris observatory in 2009, astronomers may be close to solving this mystery. Kervella noticed a large plume of gas extending outward at least six times the stellar radius indicating that the star is not shedding matter evenly in all directions.[35][31] The plume's presence, in fact, implies that the spherical symmetry of its photosphere, often observed in the infrared, is not preserved in its close environment. Asymmetries on the stellar disk had been reported many times at different wavelengths. However, due to the refined capabilities of the NACO adaptive optics on the VLT, these asymmetries have come into focus. The two mechanisms that could cause such asymmetrical mass loss, Kervella noted, were large-scale convection cells or polar mass loss, possibly due to rotation.[35] Probing deeper still with ESO's AMBER, Keiichi Ohnaka observed that the gas in the supergiant's extended atmosphere is vigorously moving up and down, creating bubbles as large as the supergiant itself, leading his team to conclude that such stellar upheaval is behind the massive plume ejection observed by Kervella.[31][36]
Evidence of circumstellar shells surrounding M supergiants was first proposed by Walter Adams and Elizabeth MacCormack in 1935 when they observed anomalies in the spectral signature of such stars and concluded that the likely cause was an expanding gaseous envelope.[90][91] In 1955, Armin Deutsch noticed in the Rasalgethi system that spectroscopic peculiarities were mysteriously occurring in the G star companion, α2 Her, from which he concluded that the whole system had to be enveloped by a circumstellar shell composed of matter being ejected by the main star, M supergiant α1 Her, and extending to at least 170 stellar radii.[90][92] In the mid 1970s, Andrew Bernat undertook a detailed analysis of four circumstellar shells, Betelgeuse, Antares, Rasalgethi and Mu Cephei, concluding that red stars dominate mass return to the Galaxy.[90]
Asymmetric shells
[edit]In addition to the photosphere, six other components of Betelgeuse's atmosphere have now been identified. Extending outward, one encounters a molecular environment otherwise known as the MOLsphere, a gaseous envelope, a chromosphere, a dust environment and two outer shells (S1 and S2) composed of carbon monoxide (CO). There is also evidence of coronal plasma in the star's extended atmosphere, a phenomenon that heretofore was not believed to exist in late stage stars off the main sequence.[25] Some of these elements are known to be asymmetric while others overlap.[10]

At about .45 stellar radii (~2–3 AU) above the photosphere, the closest membrane appears to be the molecular layer known as the MOLsphere. Studies show it to be composed of water-vapor and carbon monoxide with an effective temperature of about 1500 ± 500K.[10][37] Water-vapor had been originally detected in the supergiant's spectrum back in the 1960s with the two Stratoscope projects but had been ignored for decades. Recent studies suggest that the MOLsphere may also contain SiO and Al2O3—molecules which could explain the formation of dust particles.

Between two and seven stellar radii (~10–40 AU), astronomers have identified another region known as an asymmetric gaseous envelope composed of elemental abundances C, N and O.[10][38] The radio-telescope images taken in 1998 confirm that Betelgeuse has a dense atmosphere with a "remarkably complex structure".[39] Observations show the atmosphere to be boiling with a temperature of 3,450 ± 850K—similar to the temperature recorded on the star's surface but much lower than surrounding gas in the same region.[39][40] The VLA images also showed this lower-temperature gas progressively decreasing in temperature as it extends outward—the existence of which, although unexpected, turns out to be the most abundant constituent of Betelgeuse's atmosphere. "This alters our basic understanding of red-supergiant star atmospheres", explained Jeremy Lim, the team's leader. "Instead of the star's atmosphere expanding uniformly because of gas heated to very high temperatures near its surface, it now appears that several giant convection cells propel gas from the star's surface into its atmosphere."[39] This is the same region in which Kervella's 2009 finding of a bright plume, possibly containing CN and extending at least six photospheric radii in the southwest direction of the star, is believed to exist.[10]
The chromosphere, as mentioned earlier, was resolved in 1996 at about 2.2 times the optical disk (~10 AU) at ultraviolet wavelengths and is reported to have a temperature no higher than 5,500K.[41][10] The image was taken with the Faint Object Camera on board the Hubble Space Telescope and also revealed a bright area in the southwest quadrant of the disk. However, in 2004 observations with the STIS, Hubble's high-precision spectrometer, pointed to the existence of warm chromospheric plasma at least one arcsecond away from the star. At a distance of 197 pc, the size of the chromosphere could be 200 AU[42] The CfA team led by Alex Lobel concluded that the spatially resolved STIS spectra directly demonstrate the co-existence of warm chromospheric plasma with cool gas in Betelgeuse's circumstellar dust envelope.[42]

The first attestation of a dust shell surrounding Betelgeuse was put forth by Sutton and colleagues, who noted in 1977 that dust shells around mature stars often emit large amounts of radiation in excess of the photospheric contribution. Using heterodyne interferometry, they concluded that the red supergiant emits most of its excess beyond 12 stellar radii or roughly the distance of the Kuiper belt at 50 to 60 AU, depending on the assumed stellar radius.[10][45] Since then, there have been numerous studies done of this dust envelope at varying wavelengths yielding decidedly different results. More recent studies have estimated the inner radius of the dust shell anywhere from 0.5 to 1.0 arcseconds, or 100 to 200 AU.[46][47] What these studies point out is that the dust environment surrounding Betelgeuse is anything but static. In 1994, Danchi et al. reported that Betelgeuse undergoes sporadic dust production involving decades of activity followed by inactivity. A few years later, a group of astronomers led by Chris Skinner noticed significant changes in the dust shell's morphology in just one year, suggesting that the shell is asymmetrically illuminated by a stellar radiation field strongly affected by the existence of photospheric hotspots.[46] The 1984 report of a giant asymmetric dust shell located 1 pc (206,265 AU) from the star has not been corroborated in recent studies, although another report published the same year said that three dust shells were found extending four light years from one side of the decaying star, suggesting that, like a snake, Betelgeuse sheds its outer layers as it journeys across the heavens.[48][49]
Although the exact size of the two outer CO shells remains elusive, preliminary estimates suggest that one shell extends from about 1.5 to 4.0 arcseconds with the other expanding as far as 7.0 arcseconds.[50] Using the Jovian orbit of 5.5 AU as the "average" radius for this gargantuan star, the inner shell would extend roughly 50 to 150 stellar radii (~300 to 800 AU) with the outer one as far as 250 stellar radii (~1400 AU). With the heliopause estimated at about 100 AU, the size of this outer shell is almost fourteen times the size of the Solar System.
Supersonic bow shock
[edit]Studies since the beginning of the millennium have revealed that Betelgeuse is travelling supersonically through the interstellar medium (ISM) at a speed of 30 km per second (i.e. ~6.3 AU per year) creating a bow shock.[51] The shock is not created by the star itself, but rather a powerful stellar wind as it ejects vast amounts of gas into the ISM at a rate of 17 km/sec, heating up the material surrounding the star thereby making it visible in infrared light. Because Betelgeuse is so bright, it was only in 1997 that the bow shock was first imaged. The cometary structure is estimated to be at least 1 parsec, assuming a current distance of 643 light years.[52]
Recent 3D hydrodynamic simulations of the bow shock indicate that it is very young—less than 30,000 years old—suggesting either of two possibilities: one, that Betelgeuse moved into a region of the ISM with very different properties recently or two, that Betelgeuse itself has undergone a significant transformation as its stellar wind has changed.[53] In their 2012 paper, Mohammed et al. propose that this phenomenon was caused by Betelgeuse transitioning from a blue supergiant (BSG) to a red supergiant (RSG). In fact, in the late evolutionary stage of a star like Betelgeuse, evidence suggests that stars "may undergo rapid transitions from red to blue and vice versa on the Hertzsprung-Russell diagram, with accompanying rapid changes to their stellar winds and bow shocks."[54][55] Moreover, if future research bears out this hypothesis, Betelgeuse may well prove to have traveled close to 200,000 AU as a red supergiant scattering as much as 3 along its trajectory.
______________________________ END OF SUB-SECTION______________________________________
Circumstellar Input
[edit]1.7 The spots observed on the surface of Betelgeuse
Thanks to the pupil masking technique, Wilson et al. (1992,1997) and Buscher et al. (1990) have detected the presence of spots on the surface of Betelgeuse that represent up to 15-20% of the observed flux in the visible. The most likely origin of these asymmetries is a signature of the convection phenomenon. However, the hypotheses of inhomogeneities in the molecular layers or the presence of a transiting companion are not ruled out (Buscher et al. 1990). These observed spots are few, 2 or 3, and their characteristrics change with time (Wilson et al. 1992). Confirming previous results, Young et al. (2000) observed spots in the visible but show a fully centro-symmetric picture of Betelgeuse in the near infrared (at 1.29 m). Lately Tatebe et al. (2007) also report the observation of an asymmetry at 11.15 m located on the southern edge of the disk of Betelgeuse. In order to deepen our knowledge on RSGs, it is critical to observe spots at high angular resolution. Many unknowns remain such as their size, location, chemical composition, dynamical properties, lifetime and origin.
Circumstellar Web Articles
[edit]- NRAO 1998: A team of astronomers says that observations with the National Science Foundation's Very Large Array (VLA) radio telescope show that a neighboring bloated star has giant convective plumes propelling gas from its surface (photosphere) up into the star's atmosphere. This new information contradicts long-held ideas that such stellar atmospheres are more uniform, and may resolve questions about how the star's atmosphere attains its enormous size as well as how dust and gas is driven away from the star. Jeremy Lim of the Academia Sinica Institute of Astronomy & Astrophysics in Taiwan; Chris Carilli, Anthony Beasley, and Ralph Marson of the National Radio Astronomy Observatory (NRAO) in Socorro, NM; and Stephen White of the University of Maryland studied the red-supergiant star Betelgeuse, about 430 light-years away in the constellation Orion. They reported their findings in the April 9 issue of the scientific journal Nature. These radio-telescope images confirm that Betelgeuse -- already more than 600 times larger than our Sun -- has a dense atmosphere that extends to many times larger still than the star itself," said Lim.[39]
- SolStation: On July 29, 2009, astronomers (including Pierre Kervella and Keiichi Ohnaka) working at the European Southern Observatory announced that they had obtained the sharpest images ever of the red supergiant Betelgeuse. In their images, they detected a large plume of gas extending into space from the star's surface, which extends across a length of at least six times the diameter of the star (roughly the distance between the Sun and Neptune). The images indicate that the outer shell of the star is not shedding matter evenly in all directions, but primarily through a plume that may be ejected by large convective gas bubbles in Betelgeuse's atmosphere which is moving vigorously up and down. The convective bubbles of gas are as large as the star itself (ESO press release; Astronomy Picture of the Day; Kervella et al., 2009; and Ohnaka et al., 2009).[77]
- SolStation: Various measures, particularly those made at higher energy wavelengths where the star's outer gases are particularly opaque, suggest an enormous size (more discussion). In red light, Betelgeuse has an angular diameter of 0.054 arcseconds at 720 nm (Wilson et al., 1992), so that it would extend to 3.6 AUs into the Main Asteroid Belt within the Solar System (for a diameter of 7.2 AUs in red light). In more energetic ultraviolet light, its radius has been measured at over eight AUs out (for a diameter of 16 AUs), or more than twice that at optical wavelengths. Finally, the large radio photospheres of red giant and supergiant stars give rise to detectable thermal radio emission, so that in radio wavelengths, the star's has been found to extend beyond Saturn's orbit (more discussion). Further ultraviolet and far ultraviolet images of the star revealed a chromospheric diameter as large as 260 to 270 milli-arcseconds, or 34 to 35 AUs -- between the orbits of Neptune and Pluto (Timothy et al., 2002; and Uitenbroek et al., 1998).[77]
- SolStation: Betelgeuse is surrounded by shells of dust and gas that it has already blown off through a very strong stellar wind. There is a partial ring of dust lying out at around three times its radius, but more was found at around 650 AUs (James Kaler, 2001; and Rinehart et al., 1998). Even more dust is found around 12,000 AUs out, or 3,000 times the stellar radius. At about 36,000 AUs (or 1,000 times the stellar radius) or so, there is also abundant gas (James Kaler, 2001). In addition, there is a great, assymmetric shell of dust extending to at least 3.3 light-years or one parsec from the star (Baud et al., 1984). Although Betelgeuse is very large in diameter, astronomers believe that it contains no more than 20 Solar-masses so that the star has an average density less than air (University of Leicester Astronomy Society).[77]
- SolStation: In 1998, a team of astronomers announced that observations with the National Science Foundation's Very Large Array (VLA) radio telescope showed that the star has giant convective plumes which propel gas from its surface (photosphere) up into its atmosphere. Previous observations with the Hubble Space Telescope at ultraviolet wavelengths showed that the star's atmosphere contains very hot gas at about twice the surface temperature. The VLA images showed that there also is lower-temperature gas throughout the atmosphere. This gas is near the surface temperature at low heights and decreases in temperature progressively outwards. Although its existence was not previously suspected, this lower-temperature gas turns out to be the most abundant constituent of Betelgeuse's atmosphere. (NRAO press release). Such refinements in the images of Betelgeuse's atmosphere has been helping to resolve the mystery of how massive amounts of dust and gas are expelled from red supergiant stars, an important source of enrichment for the interstellar medium. More recent observations suggest that the relatively cool environment at lower levels allows dust grains to condense effectively, from which they can be strongly propelled in to deep space by intense radiation that carries gas with them.[77]
- CfA Press Release 2004 New observations with the Space Telescope Imaging Spectrograph (STIS), Hubble's high-precision and ultra-sensitive spectrometer, show that the warm chromosphere of Betelgeuse extends out to more than fifty times its radius in visible light, a size five times larger than the orbit of Neptune. (The chromosphere is an inner layer of a star's atmosphere, between the photosphere and the corona. The Sun's chromosphere is visible as a thin reddish line during a total solar eclipse, and extends outward for only a fraction of a solar radius.)
- ESO 2009 Sharpest views of Betelgeuse reveal how supergiant stars lose mass. Using different state-of-the-art techniques on ESO's Very Large Telescope, two independent teams of astronomers have obtained the sharpest ever views of the supergiant star Betelgeuse. They show that the star has a vast plume of gas almost as large as our Solar System and a gigantic bubble boiling on its surface. These discoveries provide important clues to help explain how these mammoths shed material at such a tremendous rate.
- Sky&Telescope 2009 Betelgeuse: A Hotheaded Superstar. Despite all the optical horsepower afforded by the Hubble Space Telescope and cutting-edge observatories on the ground, astronomers have been able to resolve the disks of only a handful of stars. One of the few successes is Betelgeuse, the enormous red supergiant marking the shoulder of Orion.
- Name YEAR
- Name YEAR
- Name YEAR
Circumstellar Source Data
[edit]- Adams MacCormack 1935 "A possible explanation of the orgitin of the abnormal lines in these giant M-type stars may be an envelope of gas surrounding the stars and exapanding with a moderate velocity."
- Schwarzschild 1975: It is presently believed that the late evolution phases of stars are greatly affected by the occurrence of mass ejection. It appears plausible - though far from certain - that this mass ejection is triggered by photospheric convection.
- Schwarzschild 1975: Early investigations of the mass loss from stars used as a starting point the theory of the solar wind, which is a clear example of mass loss, however minute, and which is believed to be basically caused by the solar subphotospheric convection. These early attempts appear, however, not to have been successful, since it has been shown (Weyman 1962) that any choice of scaling of the solar-wind model appropriate for red giants leads to a contradiction with observations regarding the circumstellar envelopes of these stars.[33]
- Bernat 1977 The first observational evidence that gas shells surround the M supergiant stars was obtained by Adams and MacCormack (1935). They noticed that the resonance lines and lines arising from low-lying levels were asymmetric and were systematically violet-shifted compared with the other lines of the spectrum. The first indication that these gas shells were vastly extendd was presented by Deutsch (1956), who observed such lines not only in the M supergiant Alpha1 Her but also in its GO III companion Alpha2 Her. Since such lines have never been obsrved in G stars, he was able to conclude that the same matter that gave rise to these lines in the spectrum of the M supergiant extended to at least 170 stellar radii -- truly a circumstellar shell. This result also demonstrated that the matter in the shell was being lost from the system, since the 10 km s-1 velocity of the matter was greater than the escape velocity at 170 stellar radii, although much less than the escape velocity at the surface of the M supergiant.
- Sutton 1977 Circumstellar dust shells around late-type stars in many cases emit large amounts of radiation near 10 microns in excess of that contributed by the central stars. Woolf and Ney (1969) suggested that this excess is characteristic of thermal emission from silicate emission from silicate greains. Other evidence for the existence of circumstellar dust grains has been found in measurements of the intrinsic polarization of visible light from these stars (Dyck et al. 1971;Forrest et al. 1975). Although work has been done to predict the density and temperature distribution of this dust, more experiemental results which measure these properties are needed.
- Baud 1984 A giant asymmetric dust shell around Betelgeuse located at 1pc.
- Roddier 1985 Not only does the visibility map show a clear departure from rotational symmetry but also its azimuthal average is found to be inconsistent with that of a stellar disk, whichever limb darkening coefficient is assumed. Two possible interpretations were discussed. One interpretation is that a large fraction of the light comes from scattering by an irregular envelope close to the stellar disk. The other is that large structures such as hot convective cells or bright prominences produce the observed effect. The data were obtained through a 90A bandwidth filter centered at 5350A (535 nm).
- Mauron 1989 New observations of atomic line scattering in the outer envelope of Betelgeuse are reported. Na I 5896-A line emission is detected for the first time using Fabry-Perot imaging and traced out to 17 arcsec from the star. The K I 7699-A line has been reobserved at offsets of 5 arcsec (E and S) and 7.5 arcsec (N) using spectroscopy with resolution 3 km/sec. The K I profiles show some differences with previously published data, probably because of an improved signal-to-noise ratio. They display asymmetries which are now in good qualitative agreement with the radiative-transfer calculations of Bernat (1976).
- Buscher 1990: High-resolution images of the M supergiant Betelgeuse in February 1989 at wavelengths of 633, 700, and 710 nm were made using the nonredundant masking method. At all these wavelengths, there is unambiguous evidence for an asymmetric feature on the surface of the star, which contributes 10-15 percent of the total observed flux. This might be due to a close companion passing in front of the stellar disk or, more likely, to large-scale convection in the stellar atmosphere.
- Huggins 1994 We report the detection of emission in the 609 micrometers 3P1 to 3P0 fine-structure line of neutral atomic carbon in the circumstellar envelope of alpha Ori, using the 10.4 m telescope of the Caltech Submillimeter Observatory. The C I line is centered at a radial velocity of +3 km/s, with an intensity of 200 mK, and a width of 30 km/s, characteristic of the expanding circumstellar envelope. The observations show that C I is an important component of the circumstellar gas. The number of C I atoms in the envelope exceeds the number of CO molecules by a factor of approximately 5, and the C I column density is large enough that significant absorption of the stellar and chromospheric spectrum can be expected at wavelengths shorter than the C I ionization edge at 1100 A. The mass-loss rate determined from our observations using the photospheric carbon abundance and assuming full recombination of carbon in the transition region is 2 x 10-6 solar mass/yr. This is in good agreement with the value determined from H I 21 cm observations and confirms that C I is a major carrier of carbon between the chromosphere and the outer envelope.
- Danchi 1994 The spatial distribution of dust around a sample of 13 well-known late-type stars has been studied with the Infrared Spatial Interferometer (ISI) located at Mount Wilson. Comprised of two movable telescopes, currently used as a heterodyne interferometer at 11.15 micrometer(s), the ISI ha S obtained visibility curves of these stars at baselines up to 13 m. This is the first detailed observational study in the mid-infrared with sufficient resolution to determine the inner radii of the dust shells of such stars. Visibility curves obtained from three-dimensional radiative transfer models of the dust shells were used to make estimates of the inner radii, temperature, and the optical depth at 11 micrometer(s) by chi2 fitting the visibility data. Results from the models are compared not only with the high spatial resolution data, but also with spectral data obtained by the Infrared Astronomy Satellite (IRAS) Low Resolution Spectrometer. For stars in which the dust is resolved, estimates of the stellar diameters and temperatures were also made. Broadly speaking, two classes of stars have been found. One class has inner radii of their dust shells very close to the photospheres of the stars themselves (3-5 stellar radii) and at a higher temperature (approximately 1200 K) than previously measured. This class includes R Leo, VX Sgr, VY CMa, IK Tau, R Aqr, o Cet, and IRC+10216. For the latter two, the visibility curves are shown to change with the luminosity phase of the star and new dust appears to form at still smaller radii during minimum luminosity. The second class of stars has dust shells with substantially larger inner radii and very little dust close to the stars, and includes alpha Ori, alpha Sco, alpha Her, chi Cyg, W Aql, and U Ori. This indicates sporadic production of dust with episodes of substantial dust production typically separated by a few decades.
- Gilliland Dupree 1996 Our imaging of Betelgeuse in the ultraviolet has opened a new chapter of stellar physics research. We highlight here a few of the potential scientific results. Measurement of the size of the apparent diameter of the disk emission coupled with a determination of the limb-darkening provides parameters to model center-to-limb behavior at other wavelengths. A proper interpretation of the interferometric measures of stellar diameters (Christou et al. 1988) depends on knowledge of the center-to-limb profile. In showing one dominant bright feature the atmosphere of Betelgeuse is dramatically different from the UV image of the Sun (the only other star with a directly imaged disk) which typically has a mottled appearance arising from many active regions. We have evidence for a totally new physical phenomenon in luminous stellar atmospheres that promises to elucidate structure and energy balanace in low gravity atmospheres. The discovery that the UV flux is dominanted by a bright feature on one hemisphere of Betelgeuse can affect the ionization structure of the nearby material. Betelgeuse has a warm chromosphere and lacks dust within 900 mas radius (Danchi et al. 1994), so the radiation field will be an important factor in determining the structure of the circumstellar envelope (Huggins et al. 1994.) Determination of the rotation vector of Betelgeuse from spatially resolved spectroscopy and/or motions of surface features may provide the link between observations of asymmetries in the envelope and circumstellar features.
- Dupree 1997 We propose continuation of spatially resolved observations of Alpha Orionis { Betelgeuse; M2 Iab} during Cycles 7 and 8 for which we were originally approved in Cycle 6 {GO 6529}. Cycle 6 observations of Betelgeuse with the FOC are scheduled for October 1996 and March 1997. Our UV images of Alpha Orionis { Betelgeuse; M2 Iab} obtained with the FOC in 1995 {Cycle 5} are the first direct images of the surface of a star other than the Sun. The continuum images reveal a greatly extended atmosphere in the ultraviolet, with diameter of approximately 125 milliarcsec, about twice that in the visible. In addition, a bright unresolved spot is present in the south-western quadrant of the stellar disk. Spectra of the Mg, ii h&k lines obtained during Cycle 5 with the GHRS show enhanced emission in the spot, spatial extent beyond the continuum image to a diameter of at least 220 mas, and a general outflow in the chromosphere. The distribution of photospheric line shifts across the stellar disk reveals the rotation of the star, and suggests the UV spot is located at one of Betelgeuse's poles. We propose here to monitor Betelgeuse twice per year during Cycles 7 and 8 obtaining FOC images, and to use STIS to acquire spectra with higher spatial resolution and larger spectral range then previously available to map motions through the atmosphere, to document its variability, and to confirm our interpretation of the spot as a propagating atmospheric shock.
- Skinner 1997 We report new mid-infrared and radio images of the M supergiant alpha Orionis. The radio images, taken with MERLIN and the Very Large Array, resolve the chromosphere of the star at a wavelength of 6 cm, showing that the radio-emitting region has between two and three times the diameter of the optical photosphere. The infrared images, taken at the UK Infrared Telescope (UKIRT) using the Berkeley Infrared Camera, show that the dust shell is resolved. Images taken one year apart show great changes in morphology, which suggests that the dust shell is being asymmetrically illuminated by a stellar radiation field that is strongly affected by the presence and evolution of spots on the stellar surface. We present a new model of the circumstellar environment of the star, which fits the infrared and radio images and the spectral energy distribution (SED), and is consistent with recently reported Hubble Space Telescope observations. The chromospheric structure is determined quantitatively and the inner dust shell structure qualitatively with this model. We find that the inner radius of the dust shell is approximately 0.5arcsec, which disagrees with a number of interferometric measurements that have resulted in an inner radius close to 1.0arcsec. We are unable to explain this difference.
- Wilson 1997 We describe a sequence of four optical interferometric observations of Betelgeuse, obtained using the nonredundant aperture mask method at the 4.2-m William Herschel Telescope on La Palma between 1994 November and 1995 January. The observations reveal complex asymmetries in the brightness distribution of the star, detected at a very high SNR. Adequate modeling of the intensity structure requires at least three bright spots superposed on the stellar disk. Changes in the relative flux and positions of the spots are evident over the 8-wk period spanning the observations. The brightness and maximum size of these features are consistent with convective surface hotspots. Simultaneous photometry of the integrated light of Betelgeuse shows a large and rapid dimming over the same period, which is not correlated with any change in the bright features. We deduce that, in this instance, the photometric variation did not result from localized convective activity associated with the spots. The interferometric data also show strong evidence for structure on a scale much larger than the photospheric diameter, which has not been present in previous observations over recent years. We attribute this new component to scattering from a newly formed dust halo, with a minimum angular diameter of approximately 0.3 arcsec.
- Lim 1998 Supergiant stars such as Betelgeuse have very extended atmospheres, the properties of which are poorly understood. Alfvén waves, acoustic waves, and radial pulsations have all been suggested as likely mechanisms for elevating these atmospheres and driving the massive outflows of gas seen in these stars: such mechanisms would heat the atmosphere from below, and there are indeed observations showing that Betelgeuse's extended atmosphere is hotter than the underlying photosphere,. Here we report radio observations of Betelgeuse that reveal the temperature structure of the extended atmosphere from two to seven times the photospheric radius. Close to the star, we find that the atmosphere has an irregular structure, and a temperature (3,450 +/- 850K) consistent with the photospheric temperature but much lower than that of gas in the same region probed by optical and ultraviolet observations. This cooler gas decreases steadily in temperature with radius, reaching 1,370 +/- 330K by seven stellar radii. The cool gas coexists with the hot chromospheric gas, but must be much more abundant as it dominates the radio emission. Our results suggest that a few inhomogeneously distributed large convective cells (which are widely believed to be present in such stars) are responsible for lifting the cooler photospheric gas into the atmosphere; radiation pressure on dust grains that condense from this gas may then drive Betelgeuse's outflow.
- Lobel Dupree 2000 A spectral analysis of the prototypical red supergiant star α Ori that is based on near-UV, optical, and near-IR high-dispersion spectra obtained between 1992 September and 1999 July with the Space Telescope Imaging Spectrograph and the Goddard High Resolution Spectrograph on the Hubble Space Telescope, the Utrecht Echelle Spectrograph, and the SoFin Echelle Spectrograph is presented. With detailed non-LTE radiative transfer calculations in spherical geometry, we model the mean conditions in the stellar chromosphere from Hα and the Mg II resonance doublet. The Hα absorption line emerges from an extended chromosphere. Temporal changes of its velocity structure are determined from detailed fits to near-UV Si I lines, and chromospheric expansion velocities around 4 km s -1 are found in 1992, whereas the chromosphere was collapsing onto the photosphere with a velocity of 5 km s -1 in 1998-1999. The Hα core depth is correlated over time with weaker depression changes seen in prominent TiO band heads that dominate the optical spectrum. From elaborate spectral synthesis calculations, we isolate unblended metal absorption lines in the near-IR and determine Teff=3500 K and log(g)=-0.5 for solar metallicity and 12+/-0.5 km s -1 for macrobroadening and vsini. Semiempirical fits yield chromospheric temperatures not in excess of 5500 K, but with long-term changes by ~400 K. The model extends over 5000 Rsolar and requires supersonic microturbulence values ranging to 19 km s -1, in strong contrast with the photospheric value of only 2 km s -1. We observe Doppler shifts of 4–8 km s -1 in the scattering cores of many double-peaked near-UV emission lines which correlate with changes in the intensity ratio of their emission components. The red emission components were much stronger in 1992, indicating a phase of enhanced chromospheric outflow, for which we determine a spherical mass-loss rate of 6×10-7 Msolar yr -1. We present a discussion of chromospheric pulsation in this massive star. Detailed modeling of the observed Mg II h and k line asymmetry is also presented. We demonstrate that a chromospheric Mn I blend strongly contributes to this puzzling asymmetry. Based in part on observations with the NASA/ESA Hubble Space Telescope obtained at the Space Telescope Science Institute, which is operated by AURA, Inc., under NASA contract NAS 5-26555.
- Tsuji 2000 We reanalyze the spectra of α Ori (M2 Iab) and μ Cep (M2 Ia) observed with the balloon-borne telescope Stratoscope II more than 35 years ago, and we confirm the presence of water in these early M supergiant stars. This identification was first proposed by the Stratoscope observers themselves (Woolf, Schwarzschild, and Rose in 1964; and Danielson, Woolf, and Gaustad in 1965), but this important discovery was overlooked for a long time without any follow-up observation. Consequently, this finding has so far had little influence on the theory of the atmosphere of red supergiant stars. A reason for this may be due to an early criticism by Wing and Spinrad, who suggested CN instead of H2O for the spectral features observed by Stratoscope II. This alternative proposition has more easily been accepted since CN has widely been observed from the Sun to red supergiants, while H2O has been observed only in very cool stars such as Mira variables. In fact, we confirm that the self-consistent photospheric model of the early M supergiants shows CN bands but no H2O band in the near-infrared. Nevertheless, we find that the contribution of CN is only minor and that H2O should be the dominant absorber for the 1.4 and 1.9 μm features on the Stratoscope spectra of α Ori and μ Cep, a conclusion opposite to that of Wing and Spinrad. The observed spectra can best be interpreted by the water gas with the column density of the order of 1020 cm-2 and temperature about 1500+/-500 K, but they cannot be originating in the photosphere. We suggest a possible presence of a gaseous component not as hot as the chromosphere but warmer than the cool expanding envelope. On the other hand, we notice that the mid-infrared pure-rotation lines of H2O recently discovered on Betelgeuse (α Ori) and Antares (α Sco) by Jennings and Sada may partly be originating in the photosphere, even though the larger part should again be nonphotospheric in origin. Thus, the presence of water possibly originating in the outer atmosphere of Betelgeuse is confirmed by the independent observation in the mid-infrared region. We now conclude that water should be an important new probe on the atmosphere of the early M supergiant stars, for which water has not been recognized as such until recently.
- Harper 2001 We have constructed a detailed mean density and temperature model for the extended outer atmosphere of the O-rich supergiant Betelgeuse (α Ori [M2 Iab]), which extends from 1.0 to 10.0 stellar radii. A one-dimensional model is based on fitting NRAO1 VLA centimeter visibility data, and two-dimensional models are constructed using the intensity contours of the 0.7 cm observations of Lim et al. As one moves in toward the star from about 10 R* the mean electron temperature increases to a value of ~=3800 K, then declines down below Teff, and then rises to photospheric values. The peak mean model temperature is less than the typical chromospheric temperatures found in previous models. Observations of Hα and chromospheric ultraviolet (UV) emission show that higher temperature components must also exist, but they do not dominate the weighted mean temperature structure. We tentatively identify the radius where the temperature distribution peaks (R~=1.45 R*) with the dominant chromospheric UV emission region and find an areal filling factor of <=1/4. In the extended semiempirical model the dominant source of electrons is from photoionized metals and is dominated by carbon. The low ionization of hydrogen leads to a dominance by H- (free-free) opacity at centimeter wavelengths. We derive simple estimates of the radio spectral indices for other similar M supergiants. We have constructed two-dimensional models to examine whether the intensity asymmetry observed at 0.7 cm is most likely to result from density or temperature variations. Adopting an elliptical two-dimensional model, a density asymmetry along the axes of symmetry would need to be 20:1. If we assume the radial wind velocity is independent of angle the integrated mass-loss rate is only a factor of ~2 greater than that derived from the one-dimensional model. However, previous Hα speckle observations that sample the same spatial regions suggest the asymmetry observed at 0.7 cm is not due to such a large-scale density asymmetry. A modest change in temperature can more easily provide the asymmetry, increasing both the opacity and the thermal source term. If the radial density structure is assumed to be the same as in 1992 September, when HST/GHRS spectra were obtained, then the Fe II wind absorption features provide an estimate of the mass-loss rate of 3.1(+/-1.3)×10-6 Msolar yr-1. This further implies that the cool material dominates the mass of the extended atmosphere and that the radio-emitting region is within the base of the outflow observed in the circumstellar layers. A simple silicate dust model is constructed and the semiempirical model suggests an onset of dust formation at R~=33 R* where Tdust~360 K. This region lies outside the semiempirical model but simple extrapolations suggest that at this radius Te~220 K, and the mean hydrogen density nH~3×106 cm-3. We address the difficult question of whether the mean thermal model based on the radio data can be consistent with the observed off-limb Hα scattering emission if inhomogeneities are present.
- Freytag 2002 Numerical radiation hydrodynamics simulations of stellar surface convection have gained a high level of reliability and perform very well in the confrontation with observations. Similar simulations of convection zones in stellar interiors are much more difficult to set up because of the huge range of time-scales to cover. Therefore, simplified models have to be used, where the results have to be carefully extrapolated to apply them to stellar interiors. Simulations of shallow surface convection zones show that the overshooting velocity fields extend far beyond the region with significant convective energy flux.
- Freytag 2002 The observed irregular brightness fluctuations of the well-known red supergiant Betelgeuse (alpha Ori, M2 Iab) have been attributed by M. Schwarzschild (1975) to the changing granulation pattern formed by only a few giant convection cells covering the surface of this giant star. The surface structure revealed by modern interferometric methods appears to be generally consistent with the explanation as large-scale granular intensity fluctuations. The interferometric data can be modeled equally well by assuming the presence of a few (up to 3) unresolved hot or cool spots on a limb-darkened disk. In an effort to improve our theoretical understanding of the Betelgeuse phenomena, we have applied a new radiation hydrodynamics code (CO5BOLD) to the problem of global convection in giant stars. For this purpose, the "local box" setup usually employed for the simulation of solar-type surface convection cannot be used. Rather, we have chosen a radically different approach: the whole star is enclosed in a cube ("star-in-a-box" setup). The properties of the stellar model are defined by the prescribed gravitational central potential and by a special inner boundary condition which replaces the unresolved core, including the source of nuclear energy production. We present current results obtained from this novel generation of 3D stellar convection simulations, proceeding from a toy model ("Mini-Sun") towards the numerically more demanding supergiant regime. We discuss the basic observational properties of Betelgeuse in the light of our best model obtained so far (T_eff = 3300 K, log g = -0.4). Finally, we describe a first attempt to investigate the interaction of the global convective flows with magnetic fields based on the kinematic approximation.
- Maeder Meynet 2000 In this article we first review the main physical effects to be considered in the building of evolutionary models of rotating stars on the Upper Main-Sequence (MS). The internal rotation law evolves as a result of contraction and expansion, meridional circulation, diffusion processes, and mass loss. In turn, differential rotation and mixing exert a feedback on circulation and diffusion, so that a consistent treatment is necessary. We review recent results on the evolution of internal rotation and the surface rotational velocities for stars on the Upper MS, for red giants, supergiants, and W-R stars. A fast rotation enhances the mass loss by stellar winds and, conversely, high mass loss removes a lot of angular momentum. The problem of the breakup or Ω-limit is critically examined in connection with the origin of Be and LBV stars. The effects of rotation on the tracks in the HR diagram, the lifetimes, the isochrones, the blue-to-red supergiant ratios, the formation of Wolf-Rayet stars, and the chemical abundances in massive stars as well as in red giants and AGB stars are reviewed in relation to recent observations for stars in the Galaxy and Magellanic Clouds. The effects of rotation on the final stages and on the chemical yields are examined, along with the constraints placed by the periods of pulsars. On the whole, this review points out that stellar evolution is not only a function of mass M and metallicity Z, but of angular velocity Ω as well.
- Maeder Meynet 2000 Several properties of massive stars with large effects of rotation and radiation are studied. For stars with shellular rotation, i.e. stars with a constant angular velocity Omega on horizontal surfaces (cf. Zahn \cite{Za92}), we show that the equation of stellar surface has no significant departures with respect to the Roche model; high radiation pressure does not modify this property. Also, we note that contrarily to some current expressions, the correct Eddington factors Gamma in a rotating star explicitely depend on rotation. As a consequence, the maximum possible stellar luminosity is reduced by rotation. We show that there are 2 roots for the equation giving the rotational velocities at break-up: 1) The usual solution, which is shown to apply when the Eddington ratio Gamma of the star is smaller than formally 0.639. 2) Above this value of Gamma, there is a second root, inferior to the first one, for the break-up velocity. This second solution tends to zero, when Gamma tends towards 1. This second root results from the interplay of radiation and rotation, and in particular from the reduction by rotation of the effective mass in the local Eddington factor. The analysis made here should hopefully clarify a recent debate between Langer (\cite{La97,La98}) and Glatzel (\cite{Gla98}). The expression for the global mass loss-rates is a function of both Omega and Gamma, and this may give raise to extreme mass loss-rates (Omega Gamma -limit). In particular, for O-type stars, LBV stars, supergiants and Wolf-Rayet stars, even slow rotation may dramatically enhance the mass loss rates. Numerical examples in the range of 9 to 120 Msun at various Teff are given. Mass loss from rotating stars is anisotropic. Polar ejection is favoured by the higher Teff at the polar caps (geff-effect), while the ejection of an equatorial ring is favoured by the opacity effect (kappa -effect), if the opacity grows fastly for decreasing Teff.
- Maeder Meynet 2000 Grids of models for rotating stars are constructed in the range of 9 to 120 Msun at solar metallicity. The following effects of rotation are included: shellular rotation, new structure equations for non-conservative case, surface distortions, increase of mass loss with rotation, meridional circulation and interaction with horizontal turbulence, shear instability and coupling with thermal effects, advection and diffusion of angular momentum treated in the non-stationary regime, transport and diffusion of the chemical elements. Globally we find that for massive stars the effects of rotation have an importance comparable to those of mass loss. Due to meridional circulation the internal rotation law Omega (r) rapidly converges, in 1-2% of the MS lifetime, towards a near equilibrium profile which then slowly evolves during the MS phase. The circulation shows two main cells. In the deep interior, circulation rises along the polar axis and goes down at the equator, while due to the Gratton-Öpik term it is the inverse in outer layers. This external inverse circulation grows in depth as evolution proceeds. We emphasize that a stationary approximation and a diffusive treatment of meridional circulation would be inappropriate. After the MS phase, the effects of core contraction and envelope expansion dominate the evolution of the angular momentum. The surface velocities decrease very much during the MS evolution of the most massive stars, due to their high mass loss, which also removes a lot of angular momentum. This produces some convergence of the velocities, but not necessarily towards the break-up velocities. However, stars with masses below ~ 12 Msun with initially high rotation may easily reach the break-up velocities near the end of the MS phase, which may explain the occurrence of Be-stars. Some other interesting properties of the rotational velocities are pointed out. For an average rotation, the tracks in the HR diagram are modified like a moderate overshoot would do. In general, an average rotation may increase the MS lifetime up to about 30%; for the helium-burning phase the effects are smaller and amount to at most 10%. From plots of the isochrones, we find that rotation may increase the age estimate by about 25% in general. However, for stars with M >~ 40 Msun and fast rotation, a bluewards ``homogeneous-like track, with important He- and N-enrichments, may occur drastically affecting the age estimates for the youngest clusters. Rotation also introduces a large scatter in the mass-luminosity relation: at the same log g_eff and log T_eff, differences of masses by 30% may easily occur, thus explaining what still remains of the alleged mass discrepancy. Rotation also brings significant surface He- and N-enhancements, they are higher for higher masses and rotation. While it is not difficult to explain very fast rotators with He- and N-excesses, the present models also well account for the many OB stars exhibiting surface enrichments and moderate or low rotation, (cf. Herrero et al. \cite{He92}, \cite{He20}). These stars likely result from initially fast rotators, which experienced mixing and lost a lot of angular momentum due to enhanced mass loss. The comparison of the N-excesses for B- and A-type supergiants supports the conclusion by Venn (\cite{ve95a}, \cite{Ve99}), that these enrichments mostly result from mixing during the MS phase, which is also in agreement with the results of Lyubimkov (\cite{Lyu96}).
- Maeder Meynet 2003 Rotation affects all the outputs of the evolution and nucleosynthesis of massive stars. We discuss the evolution of the rotational velocities, the internal Ω-gradients, the tracks in the H-R diagram, the age determinations, the evolution of the surface N/C abundance ratios, the B/R number ratios of blue to red supergiants and the lifetimes in the WR stages.
- Lobel 2004 We present spatially resolved spectra observed with HST-STIS of the upper chromosphere and dust envelope of Alpha Orionis (M2 Iab). In the fall of 2002 a set of five high-resolution near-UV spectra was obtained by scanning at intensity peak-up position and four off-limb target positions up to one arcsecond, using a small aperture, to investigate the thermal conditions and flow dynamics in the outer atmosphere of this important nearby cool supergiant star. Based on Mg II h & k, Fe II 2716 A, C II 2327 A, and Al II ] 2669 A emission lines we provide the first evidence for the presence of warm chromospheric plasma at least 1 arcsecond away from the star at ~40 R* (1 R*~700 Rsun). The STIS spectra reveal that Betelgeuse's upper chromosphere extends far beyond the circumstellar H alpha envelope of ~5 R*, determined from previous ground-based imaging. The flux in the broad and self-absorbed resonance lines of Mg II decreases by a factor of ~700 compared to the flux at chromospheric disk center. We observe strong asymmetry changes in the Mg II h and Si I resonance line profiles when scanning off-limb, signaling the outward acceleration of gas outflow in the upper chromosphere. From the radial intensity distributions of Fe I and Fe II emission lines we determine the radial non-LTE iron ionization balance. We compute that the local kinetic gas temperatures of the warm chromospheric gas component in the outer atmosphere exceed 2600 K, when assuming local gas densities of the cool gas component we determine from radiative transfer models that fit the 9.7 um silicate dust emission feature. The spatially resolved STIS spectra directly demonstrate that warm chromospheric plasma co-exisists with cool gas in Betelgeuse's circumstellar dust envelope.
- Perrin 2004: Because of their large luminosity supergiant stars have extended atmospheres, with resultant low surface gravity and large pressure scale height. The cool supergiants are usually slightly variable in brightness, color, polarization, and radial velocity, suggestive of significant dynamic and radiative activity and inhomogeneities. However, the variability is small enough that static model atmospheres are normally considered adequate for abundance studies. Owing to the very extended atmospheres, and in some cases circumstellar shells, it is difficult to determine even some very basic parameters for these stars. Here, we address the question of the effective temperatures. The near infrared wavelength regime offers several advantages for the study of cool supergiants - it is less obscured by scattering and line blanketing (problems in the visible) and by dust (detected in the mid-infrared). Alpha Orionis is a bright, prototypical example of the cool supergiant class. It is classified M1-2Ia-Iab, which places it firmly in the supergiant category. An infrared excess and extended dust shell confirm substantial mass loss. Orionis has been observed several times with high angular resolution techniques and in several optical bandpasses. The derived angular diameters are plotted against wavelength in Fig. 1. The observed angular diameters are significantly larger in the visible, decrease to a minimum in the near-infrared, and then increase again in the mid-infrared. Some surface structure, similar to large spots, has been seen at several wavelengths in the red (Tuthill et al. 1997; Young et al. 2000). These spots have not been seen at other nearby wavelengths.
- Harper 2009 Carbon monoxide has been detected in Betelgeuse's ultraviolet (electronic), infrared (vibrational), and mm-radio (rotational) spectra, but its spatial distribution has remained elusive. We have obtained new mm-radio interferometry (CARMA), infrared spatially-resolved spectra (Phoenix/Gemini-South), and we have performed non-LTE simulations to help establish the spatial location of the S1 (V = 10 km/s) and S2 (V = 17 km/s) CO shells. The strong CO Fourth-Positive ultraviolet scattering signature is apparent in the HST GHRS G140L spectrum and the S1 and S2 shells account for much of the UV opacity. Phoenix spectra reveal that the low velocity S1 shell is present at 1.5 arcsec West of the star and extends out to ~4 arcsec, and its mm-radio emission appears to originate within an 6 arcsec radius. The interpretation of the S2 shell is less clear. The Phoenix spectra tentatively suggest that the S2 shell extends to ~7 arcsec. The CARMA channel maps show an additional strong narrow emission component 5 arcsec from the star, but the spectra do not show all the expected S1 and S2 signatures. Future CARMA observations should help to disentangle the signature of the S1 and S2 shells.
- Ridgeway 2009 Mass is constantly being recycled in the universe. One of the most powerful recycling paths is via stellar mass-loss. All stars exhibit mass loss with rates ranging from ~10(-14) to 10(-4) M(sun) yr-1, depending on spectral type, luminosity class, rotation rate, companion proximity, and evolutionary stage. The first generation of stars consisted mostly of hydrogen and helium. These shed material - via massive winds, planetary nebulae and supernova explosions - seeding the interstellar medium with heavier elements. Subsequent generations of stars incorporated this material, changing how stars burn and providing material for planet formation. An understanding of mass loss is critical for modeling individual stars as well as answering larger astrophysical questions. Understanding mass loss is essential for following the evolution of single stars, binaries, star clusters, and galaxies. Mass loss is one of our weakest areas in the modeling of fundamental stellar processes. In large part this is owing to lack of confrontation with detailed observations of stellar photospheres and the mass-loss process. High resolution optical imagery with telescope arrays is beginning to provide these data and, combined with spectroscopy and broad infrared and sub-mm coverage, supporting more sophisticated models on fast computers and promising a new era in mass-loss studies.
- Haubois 2009 Located in the Orion constellation, Betelgeuse (Orionis) is a red supergiant (hereafter RSG) of spectral type M2Iab. It is one of the brightest stars at optical wavelengths and has the second biggest angular diameter (43 mas, Perrin et al. 2004) after R Doradus (Bedding et al. 1997). Classified as semi-regular, it shows periodicity in its brightness changes, accompanied or sometimes interrupted by various irregularities (Guinan 1984). According to a recent reanalysis of a Hipparcos satellite dataset, its distance is now estimated at pc (Harper et al. 2008). The observations up to now have identified at least 7 components of the complex Betelgeuse atmosphere: two outer shells, a dust environment, a chromosphere, a gaseous envelope, a molecular shell also known as MOLsphere (Tsuji 2000a), and finally the photosphere. Some of these components are not symmetric, and some are overlapping.
- Kervella 2009: Context. Betelgeuse is one the largest stars in the sky in terms of angular diameter. Structures on the stellar photosphere have been detected in the visible and near-infrared as well as a compact molecular environment called the MOLsphere. Mid-infrared observations have revealed the nature of some of the molecules in the MOLsphere, some being the precursor of dust. Aims. Betelgeuse is an excellent candidate to understand the process of mass loss in red supergiants. Using diffraction-limited adaptive optics (AO) in the near-infrared, we probe the photosphere and close environment of Betelgeuse to study the wavelength dependence of its extension, and to search for asymmetries. Methods. We obtained AO images with the VLT/NACO instrument, taking advantage of the ``cube mode of the CONICA camera to record separately a large number of short-exposure frames. This allowed us to adopt a ``lucky imaging approach for the data reduction, and obtain diffraction-limited images over the spectral range m in 10 narrow-band filters. Results. In all filters, the photosphere of Betelgeuse appears partly resolved. We identify an asymmetric envelope around the star, with in particular a relatively bright ``plume extending in the southwestern quadrant up to a radius of approximately six times the photosphere. The CN molecule provides an excellent match to the 1.09 m bandhead in absorption in front of the stellar photosphere, but the emission spectrum of the plume is more difficult to interpret. Conclusions. Our AO images show that the envelope surrounding Betelgeuse has a complex and irregular structure. We propose that the southwestern plume is linked either to the presence of a convective hot spot on the photosphere, or to the rotation of the star.
- Kervella 2009: Several mysteries remain to be solved for such an object among which the structure of its convection and the mechanism of its mass loss. Similarities with Mira stars have been noted, including a significant thermal infrared excess, indicative of the presence of circumstellar dust. An important difference is that Betelgeuse is not subject to regular large amplitude pulsations which could levitate material high enough above the atmosphere where dust could form and be blown away by radiation pressure. The reason for the extension of the atmosphere is still unclear and another mechanism for dust formation and mass loss needs to be invoked. A careful scrutiny of the object from the photospheric scale up to the distance in its circumstellar envelope (CSE) where dust can condense is the path to understanding this prototypical and enigmatic star. It is also known from interferometric observations in the thermal infrared domain by Bester et al. (1996) that Betelgeuse experiences episodic mass loss. Our new NACO observations aim at probing the close environment of Betelgeuse to etablish a link between the photosphere and mass loss.
- Kervella 2009: Origin of the southwestern plume. The presence of the bright southwestern plume detected with NACO implies that the spherical symmetry observed on the infrared photosphere of Betelgeuse is not preserved in its close environment. Among the plausible mechanisms to explain its presence, two appear as promising: convection and rotation. Following the proposal by Schwarzschild (1975) and the simulation results by Freytag & Höfner (2008), a first hypothesis is that the very large convection cells enhance locally the mass-loss from the star, creating the observed plumes of molecules. However, although many studies have shown that the photosphere of Betelgeuse presents a significant degree of asymmetry, variable with time, the link with the chromosphere and the envelope remains to be established. A second hypothesis is that mass loss may be enhanced above the polar cap of the star, creating the southwestern plume of Betelgeuse. This possibility is supported by the HST imaging and spectroscopy by Uitenbroek et al. (1998), as it reveals the presence of a hot spot that could be located at the pole of the star. An interaction between this hot spot and the extended chromosphere of the star could result in a preferential production of molecules above the pole and the creation of a plume.
- Ohnaka 2009 Aims: We present spatially resolved, high-spectral resolution K-band observations of the red supergiant Betelgeuse (α Ori) using AMBER at the Very Large Telescope Interferometer (VLTI). Our aim is to probe inhomogeneous structures in the dynamical atmosphere of Betelgeuse. Methods: Betelgeuse was observed in the wavelength range between 2.28 and 2.31 μm with VLTI/AMBER using baselines of 16, 32, and 48 m. The spectral resolutions of 4800-12 000 allow us to study inhomogeneities seen in the individual CO first overtone lines. Results: Spectrally dispersed interferograms have been successfully obtained in the second, third, and fifth lobes, which represents the highest spatial resolution (9 mas) achieved for Betelgeuse. This corresponds to five resolution elements over its stellar disk. The AMBER visibilities and closure phases in the K-band continuum can be reasonably fitted by a uniform disk with a diameter of 43.19 ± 0.03 mas or a limb-darkening disk with 43.56 ± 0.06 mas and a limb-darkening parameter of (1.2 ± 0.07) × 10-1. These AMBER data and the previous K-band interferometric data taken at various epochs suggest that Betelgeuse seen in the K-band continuum shows much smaller deviations from the above uniform disk or limb-darkened disk than predicted by recent 3-D convection simulations for red supergiants. On the other hand, our AMBER data in the CO lines reveal salient inhomogeneous structures. The visibilities and phases (closure phases, as well as differential phases representing asymmetry in lines with respect to the continuum) measured within the CO lines show that the blue and red wings originate in spatially distinct regions over the stellar disk, indicating an inhomogeneous velocity field that makes the star appear different in the blue and red wings. Our AMBER data in the CO lines can be roughly explained by a simple model, in which a patch of CO gas is moving outward or inward with velocities of 10–15 km s-1, while the CO gas in the remaining region in the atmosphere is moving in the opposite direction at the same velocities. Also, the AMBER data are consistent with the presence of warm molecular layers (so-called MOLsphere) extending to 1.4-1.5 Rstar with a CO column density of 1 × 1020 cm-2. Conclusions: Our AMBER observations of Betelgeuse are the first spatially resolved study of the so-called macroturbulence in a stellar atmosphere (photosphere and possibly MOLsphere as well) other than the Sun. The spatially resolved CO gas motion is likely to be related to convective motion in the upper atmosphere or intermittent mass ejections in clumps or arcs.
- Lobel 2010 We present modeling research work of the winds and circumstellar environments of a variety of prototypical hot and cool massive stars using advanced radiative-transfer calculations. This research aims at unraveling the detailed physics of various mass-loss mechanisms of luminous stars in the upper portion of the H-R diagram. Very recent 3D radiative-transfer calculations, combined with hydrodynamic simulations, show that radiatively-driven winds of OB supergiants are structured due to large-scale density and velocity fields caused by rotating bright spots at the stellar equator. The mass-loss rates computed from matching Discrete Absorption Components (DACs) in IUE observations of HD 64760 (B Ib) do not reveal appreciable changes from the rates of unstructured (smooth) wind models. Intermediate yellow supergiants (such as the yellow hypergiant ρ Cas, F–G Ia0), on the other hand, show prominent spectroscopic signatures of strongly increased mass-loss rates during episodic outbursts that cause dramatic changes of the stellar photospheric conditions. Long-term high-resolution spectroscopic monitoring of cool hypergiants near the Yellow Evolutionary Void reveals that their mass-loss rates and wind-structure are dominated by photospheric eruptions and large-amplitude pulsations that impart mechanical momentum to the circumstellar environment by propagating acoustic (shock) waves. In massive red supergiants, however, clear evidence for mechanical wave propagation from the sub-photospheric convection zones is lacking, despite their frequently observed spectroscopic and photometric variability. Recent spatially resolved HST-STIS observations inside Betelgeuse's (M Iab) very extended chromosphere and dust envelope show evidence of warm chromospheric gas far beyond the dust-condensation radius of radiative-transfer models. Models for these long-term spectroscopic observations demonstrate that the chromospheric pulsations are not spherically symmetric. The STIS observations point to the importance of mechanical wave propagation for heating and sustaining chromospheric conditions in the extended winds of red supergiants.
- Petit 2010: Betelgeuse, a boiling and magnetic supergiant star. An international research team, lead by French astrophysicists from the Laboratoire d'Astrophysique de Toulouse-Tarbes, has detected a magnetic field at the surface of the supergiant star Betelgeuse. This observational result, published in the journal Astronomy & Astrophysics, demonstrates that, in spite of the theoretical framework usually proposed to account for the magnetism of astrophysical bodies like the Earth or the Sun, the rotation of cosmic objects is not a necessary ingredient to trigger the efficient generation of a magnetic field. According to a scenario elaborated more than half a century ago, the rotation of stars like the Sun produces huge flows of ionized material in their internal layers. These large-scale flows trigger a dynamo mechanism causing the continuous generation of their magnetic field. This process, called a "large-scale dynamo", is generally invoked to describe the solar magnetic cycle, which is particularly spectacular during solar eruptive phases. Yet, even when the Sun is having a temporary respite in its magnetic firework, for instance during the last, unusually long activity minimum that recently ended, our star still hosts a surface magnetic field. The origin of this residual magnetism, which seems to be unaffected by the solar cycle, is still a disputed question among astronomers. The key of this enigma may be hidden in supergiant stars, a class of objects of which Betelgeuse is one of the most famous members. With about 15 times the solar mass, 1,000 solar radii and a luminosity 100,000 times higher than the Sun's, Betelgeuse is a star reaching the end of its life while burning the last remaining nuclear fuel at its disposal before exploding as a supernova. In addition, another physical parameter of Betelgeuse is differing from the solar case : its rotation is extremely slow. It takes probably several years for Betelgeuse to complete a full rotation, against barely one month for the Sun. This situation seems inadequate to allow for the onset of a large-scale dynamo. However, observations collected with the NARVAL instrument at Telescope Bernard Lyot (Pic du Midi Observatory, France) reveal a weak polarization level in the light emitted by Betelgeuse : an observational clue unveiling the presence of a weak magnetic field at the surface of the star. This observation is therefore demonstrating that a fast rotation is not a necessary ingredient for the efficient production of a magnetic field. Supergiant stars may use another trick : vigorous convective motions, similar to a continuous boiling, are evacuating the huge amount of energy released in the stellar core by nuclear reactions. Observations obtained at Pic du Midi suggest that this continuous agitation is able, in itself, to generate the stellar magnetic field, through "small-scale" dynamo processes operating on the same scale as the convective cells. Since the Sun itself is exhibiting turbulent motions in its outer layers, it could very well be able to host a similar type of small-scale dynamo, that could be (at least partly) responsible for its residual magnetism during activity minima.
Supersonic Bow Shock
[edit]Studies since the beginning of the millennium have revealed that Betelgeuse is travelling supersonically through the interstellar medium (ISM) at a speed of 30 km per second (i.e. ~1 billion km per year) creating a bow shock. The shock is not created by the star itself, but rather a powerful stellar wind as it ejects vast amounts of gas into the ISM at a rate of 17 km/sec, heating up the material surrounding the star thereby making it visible in infrared light. Because Betelgeuse is so bright, it was only in 1997 that the bow shock was first successfully imaged. The cometary structure is estimated to be 3 light-years wide.
Recent 3D hydrodynamic simulations of the bow shock indicate that it is very young, less than 30,000 years old, suggesting either of two possibilities: one, that Betelgeuse moved into a region of the ISM with very different properties recently or two, that Betelgeuse itself has undergone a significant transformation as its stellar wind has changed. In their 2012 paper, Mohammed et al. propose that this phenomenon was caused by Betelgeuse transitioning from a blue supergiant (BSG) to a red supergiant (RSG). In fact, in the late evolutionary stage of a star like Betelgeuse, recent evidence suggests that stars "may undergo rapid transitions from red to blue and vice versa on the Hertzsprung-Russell diagram, with accompanying rapid changes to their stellar winds and bow shocks."
Bow Shock Articles
[edit]Bow Shock Research
[edit]- Noriega 1997 We present new High Resolution IRAS (HiRes) images at 60 and 100 \mum of a bow shock or shell with a mean radius of 6arcmin surrounding Betelgeuse (alpha Orionis). The outermost section of this structure is at ~ 7arcmin which corresponds to a physical scale of 0.8 pc at a distance of 400 pc. The images were made by applying a relative Burg entropy maximization algorithm to suppress the ringing around bright sources and allow the detection of nearby low surface brightness features. The shell is relatively narrow at 60 \mum (at the 1arcmin HiRes resolution), asymmetric and oriented along the star's direction of motion. We propose that the shell is confined by the ram pressure of the interstellar medium. Using a shell flux of 110 Jy (at 60 \mum) within a radius of 7.arcmin5 we estimate mass of 0.14 \msol for the shell.
- Ueta 2009 Recent far-infrared mapping of mass-losing stars by the AKARI Infrared Astronomy Satellite and Spitzer Space Telescope have suggested that far-infrared bow shock structures are probably ubiquitous around these mass-losing stars, especially when these stars have high proper motion. Higher spatial resolution data of such far-infrared bow shocks now allow detailed fitting to yield the orientation of the bow shock cone with respect to the heliocentric space motion vector of the central star, using the analytical solution for these bow shocks under the assumption of momentum conservation across a physically thin interface between the stellar winds and interstellar medium (ISM). This fitting analysis of the observed bow shock structure would enable determination of the ambient ISM flow vector, founding a new technique to probe the 3-D ISM dynamics that are local to these interacting systems. In this review, we will demonstrate this new technique for three particular cases, Betelgeuse, R Hydrae, and R Cassiopeiae.
- Mackey 2012 A significant fraction of massive stars are moving supersonically through the interstellar medium (ISM), either due to disruption of a binary system or ejection from their parent star cluster. The interaction of their wind with the ISM produces a bow shock. In late evolutionary stages these stars may undergo rapid transitions from red to blue and vice versa on the Hertzsprung-Russell diagram, with accompanying rapid changes to their stellar winds and bow shocks. Recent three-dimensional simulations of the bow shock produced by the nearby runaway red supergiant (RSG) Betelgeuse, under the assumption of a constant wind, indicate that the bow shock is very young (<30, 000 years old), hence Betelgeuse may have only recently become an RSG. To test this possibility, we have calculated stellar evolution models for single stars which match the observed properties of Betelgeuse in the RSG phase. The resulting evolving stellar wind is incorporated into two-dimensional hydrodynamic simulations in which we model a runaway blue supergiant (BSG) as it undergoes the transition to an RSG near the end of its life. We find that the collapsing BSG wind bubble induces a bow shock-shaped inner shell around the RSG wind that resembles Betelgeuse's bow shock, and has a similar mass. Surrounding this is the larger-scale retreating bow shock generated by the now defunct BSG wind's interaction with the ISM. We suggest that this outer shell could explain the bar feature located (at least in projection) just in front of Betelgeuse's bow shock.
- Mohamed 2012 Betelgeuse, the bright, cool red supergiant in Orion, is moving supersonically relative to the local interstellar medium. The star emits a powerful stellar wind which collides with this medium, forming a cometary structure, a bow shock, pointing in the direction of motion. We present the first 3D hydrodynamic simulations of the formation and evolution of Betelgeuse's bow shock. The models include realistic low temperature cooling and cover a range of plausible interstellar medium densities and stellar velocities between 0.3 - 1.9 cm-3 and 28 – 73 km/s. We show that the flow dynamics and morphology of the bow shock differ substantially due to the preferential growth of Rayleigh-Taylor or Kelvin-Helmholtz instabilities in the models. The former dominate the models with slow stellar velocities resulting in a clumpy bow shock sub-structure, whereas the latter produce a smoother, more layered sub-structure in the fast models. If the mass in the bow shock shell is low, as seems to be implied by the AKARI luminosities (~0.003 Msun), then Betelgeuse's bow shock is very young and is unlikely to have reached a steady state. The circular nature of the bow shock shell is consistent with this conclusion. Thus, our results suggest that Betelgeuse entered the red supergiant phase only recently.
- Name YEAR
- Name YEAR
Approaching Supernova
[edit]- Sub-Section Purpose: Supernova, Type, When?
Need to handle all this speculation that Betelgeuse is going to blow up soon. Sky & Telescope says "anytime" in the next million years. In stellar time, that's soon.
Fate Existing Language
[edit]The future fate of Betelgeuse depends on its mass; as it probably contains more than 15 solar masses, it will continue to burn and fuse elements until its core is iron, at which point Betelgeuse will explode as a type II supernova. During this event the core will collapse, leaving behind a neutron star remnant some 20 km in diameter.[57]
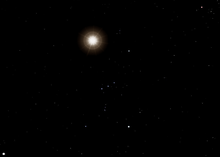
Betelgeuse is already old for its size class and will explode relatively soon compared to its age of several million years.[93] At the current distance of Betelgeuse from the Earth, such a supernova explosion would be the brightest recorded; outshining the Moon in the night sky and becoming easily visible in broad daylight.[93] Professor J. Craig Wheeler of The University of Texas at Austin predicts the supernova will emit 1053 ergs of neutrinos, which will pass through the star's hydrogen envelope in around an hour, then reach the solar system several centuries later. Since its rotational axis is not pointed toward the Earth, Betelgeuse's supernova is unlikely to send a gamma ray burst in the direction of Earth large enough to damage Earth's ecosystems.[94] The flash of ultraviolet radiation from the explosion will be weaker than the ultraviolet output of the Sun.
The supernova would brighten to an apparent magnitude of –12 over a two-week period, then remain at that intensity for two or three months before rapidly dimming. The year following the explosion, radioactive decay of cobalt to iron will dominate emission from the supernova remnant, and the resulting gamma rays will be blocked by the expanding envelope of hydrogen. If the neutron star remnant became a pulsar, then it might produce gamma rays for thousands of years.[95]
Fate Source Data
[edit]Star system
[edit]In 1985, Margarita Karovska, in conjunction with other astrophysicists at the Harvard–Smithsonian Center for Astrophysics, announced the discovery of two close companions orbiting Betelgeuse. Analysis of polarization data from 1968 through 1983 indicated that Alpha Ori had a close companion with a periodic orbit of about 2.1 years. The team realized that the observed polarization could be caused by a systemic asymmetry created by the close companion orbiting Alpha Ori inside its extended dust envelope. Using speckle interferometry, the team concluded that the closer of the two companions was located at 0.06 ± 0.01 arcseconds from the main star with a position angle of 273 degrees. The more distant companion was estimated at 0.51 ± 0.01" with a PA of 278 degrees. The magnitude differences with respect to the primary, measured at 656.3 (Hα) and 656.8 nm (red continuum), were 3.4 and 3.0 for the close component and 4.6 and 4.3 for the distant component.[18][19]

In the years that followed, different teams of astronomers began to monitor the data in the hope of obtaining additional confirmation. In 1987, Andrea Dupree made the following observation: "Periastron of the recently discovered close optical companion to Alpha Ori is predicted to be 1986.7; detection of atmospheric disturbances similar to those found subsequent to the last periastron (~ 1984.6) would give strong support to the presence of a companion."[96] However it appears that such detection never materialized. Rather, in 1990, David F. Buscher, John E. Baldwin and a team of collaborators from the Cavendish Astrophysics Group made a number of high-resolution images of the supergiant at wavelengths of 633, 700, and 710 nm using the nonredundant masking method. At all these wavelengths, they remarked, there was unambiguous evidence for an asymmetric feature on the surface of the star, which contributed 10-15 percent of the star's total observed flux. Their conclusion was that such a phenomenon could be caused by a close companion passing in front of the stellar disk, differential photospheric brightening due to the effects of stellar rotation or the more likely scenario of "large-scale convection in the stellar atmosphere" as suggested by Schwarzschild.[61]
Two years later in 1992, the Cavendish colleagues published another paper, this time under the helm of Richard. W. Wilson, noting that the brightness features on the surface of Betelgeuse appear to be "too bright to be associated with a passage of the suggested companions in front of the red giant." They also noticed that these features were fainter at the 710 than at 700 nm, by a factor of 1.8, indicating that they would have to reside within the molecular atmosphere of the star.[20]
That same year, Karovska published a new paper reconfirming her and her colleagues' interpretation of the data, but also noting that "the correlation between the calculated position angles of the companion and the measured position angles of the asymmetries suggests that there is a possible connection between the asymmetries and the companion. The asymmetry in the images of α Ori could be caused by the unresolved companion, by tidal distortion of the supergiant's atmosphere, or possibly by an unresolved bright spot on the stellar surface facing the companion. To determine the nature of the companion (which presently remains a puzzle), it is crucial to obtain further speckle observations using large aperture telescopes, coordinated with other ground-based observations and the observations from space."[21]
Since then, researchers turned their attention to analyzing the intricate dynamics of the star's extended atmosphere and little else has been published on the possibility of orbiting companions. As the decade unfolds and new technologies are brought to unraveling the star's enigmatic past, we will likely see conclusive evidence, one way or another, of any potential star system. Given the planned capabilities of the upcoming Gaia mission, a confirmation could occur any time after the mission's scheduled launch in December 2012.
System Source Data
[edit]- AAVSO 2000: Speckle Interferometry reveals dust and companions around Betelgeuse (1985). Francois and Claude Roddier constructed an image of Alpha Orionis and found indications of dust close to the red supergiant star. Their data were gathered in 1980 with an interferometer and the Canada-France-Hawaii Telescope. To explain Betelgeuse's lopsided appearance, they favor the idea that a large fraction of the recorded light comes from an irregular dust envelope close to the stellar disk. Details concerning their work are reported in Astrophysical Journal Letters for August 1, 1985. However, dust is not the only thing surrounding Betelgeuse. Margarita Karovska, an associate of the Roddiers' now based at the Harvard-Smithsonian Center for Astrophysics, announced in 1985 that the famous red supergiant has two close companions. Her results are based on speckle interferometry work done in the early 1980s at Kitt Peak, Arizona, and Cerro Tololo, Chile. It revealed that the inner of the two stars orbits Betelgeuse every two years or so at a mean distance of about 5 astronomical units and little is known about the outer companion except that it lies some 40 or 50 astronomical units from Betelgeuse (December, 1985, Sky and Telescope).[28]
- Karovska 1985:Analysis of polarization data from 1968 through 1983 indicates that they are consistent with the existenc of a close companion to Alpha Ori, with an eccentric orbit having a period of about 2.2 years. Assuming the mas of Alpha Ori is 15-20 times solar, the semi-major axis of the orbit is only about 2.5 times the radius of Alpha Ori.
- Karovska 1986: Detection of two close optical companions to the red supergiant Alpha Ori was accomplished in 1983 November on the Steward Observatory 2.25 telescope. A new two-dimensional photon counting camera (the PAPA detector) was used for data recording, and speckle imaging was used for image reconstruction. The closer of the two sources is located at 0".06 0".01 from Alpha Ori (P.A. = 273 degrees), the more distant at 0.51" 0.01" (P.A. = 278 degrees). The magnitude differences with respect to the primary, measured at 656.3 (H alpha) and 656.8 nm (red continuum) are 3.4 and 3.0 for the close source and 4.6 and 4.3 for the distant source respectively. This observation confirms the reality of the two sources which has been reported in previous work. Our analysis favors an interpretation in which the two optical sources are stellar companions to Alpha Ori. Strong support for the existence of the close stellar companion was found in the polarization data obtained by different observers for Alpha Ori. A periodicity of ~2.1 yr was found in the time-dependent variations in the position angle of the plane of polarization, which appear to reflect the orbital motion of the close companion. The observed polarization can be interpreted as being due to a sytemic asymmetry created by the close companion orbiting Alpha Ori inside its extended dust envelope.
- Dupree 1986: We propose to continue the multifrequency monitoring of Alpha Orionis (Betelgeuse), for the next two years. This will enable spectra to be obtained of the decay of chromospheric activity through the light minimum of the 5.8 year period and the radial velocity maximum (1987.04). Continuous observations with IUE for the next two years will provide a carefully constructed homogeneous data set covering almost one cycle of the 5.8 year photometric period. Periastron of the recently discovered close optical companion to Alpha Ori is predicted to be 1986.7; detection of atmospheric disturbances similar to those found subsequent to the last periastron (~ 1984.6) would give strong support to the presence of a companion.
- Dupree 1987: We propose to continue the multifrequency monitoring of Alpha Orionis (Betelgeuse), for the next two years. This will enable spectra to be obtained of the decay of chromospheric activity through the light minimum of the 5.8 year period and the radial velocity maximum (1987.04). Continuous observations with IUE for the next two years will provide a carefully constructed homogeneous data set covering almost one cycle of the 5.8 year photometric period. Periastron of the recently discovered close optical companion to Alpha Ori is predicted to be 1986.7; detection of atmospheric disturbances similar to those found subsequent to the last periastron (~ 1984.6) would give strong support to the presence of a companion.
- Dupree 1988: Our discovery (Dupree et al. 1987) of a 420-day periodic variation in the radiations from Alpha Ori (Betelgeuse) was made possible by dedicated frequent observations with the IUE satellite in conjunction with contemporaneous ground based photometric and spectroscopic observations, Periodic photospheric pulsations are the most likely explanation of these variations. The identification of pulsations in the atmosphere of Alpha Ori is important for they appear to be the fundamental pulsation mode of the star and can provide the long-sought mechanism to heat and to extend the atmosphere of the star, and help to initiate the mass flow from the supergiant. We propose to continue the multifrequency monitoring of Alpha Orionis (Betelgeuse), for the next two years in order a) to seek evidence for the secondary period of -1 6 years which is hinted at in the current measurements, b) to establish the phase delay between photospheric and chromospheric modulation, c) to evaluate the stability of the 420-day period and d) to complement the precision radial velocity measures being carried out at Kitt Peak that recently confirmed our conclusion of pulsation in the star's atmosphere. Continuous observations with IUE for the next two years will provide a carefully constructed homogeneous data set for a well-studied star covering one cycle of the 5.8 year photometric period. Additionally, we propose to introduce Mu Cep, another bright M supergiant, into the program.
- Dupree 1989: Our discovery (Dupree et al. 1987) of a 420-day periodic variation in the radiations from Alpha Ori (Betelgeuse) was made possible by dedicated frequent observations with the IUE satellite in conjunction with contemporaneous ground based photometric and spectroscopic observations. Periodic photospheric pulsations are the most likely explanation of these variations. The identification of pulsations in the atmosphere of Alpha Ori is important for they appear to be the fundamental pulsation mode of the star and can provide the long-sought mechanism to heat and to extend the atmosphere of the star, and help to initiate the mass flow from the supergiant. We propose to continue the multifrequency monitoring of Alpha Orionis (Betelgeuse), for the next, two years in order a) to seek evidence for the secondary period of ~ 6 years which is hinted at in the current measurements, b) to establish the phase delay between photospheric and chromospheric modulation, c) to evaluate the stability of the 420-day period and d) to complement the precision radial velocity measures being carried out at Kitt Peak that recently confirmed our conclusion of pulsation in the star's atmosphere. Continuous observations with IUE for the next two years will provide a carefully constructed homogeneous data set for a well-studied star covering one cycle of the 5.8 year photometric period. Additionally, we propose to introduce mu Cep, another bright M supergiant, into the program.
- Dupree 1990: Our discovery of a 420-day periodic variation in the radiations from Alpha Orionis (Betelgeuse) was made possible by dedicated frequent observations with the IUE satellite in conjunction with contemporaneous ground-based photometric and spectroscopic observations. Periodic photospheric pulsations are the most likely explanation of the photometric variations, and this IUE result has recently been confirmed by measured velocity variations in the photosphere. Identification of pulsations in the atmosphere of Alpha Ori is important for they appear to be the fundamental pulsation mode of the star, and can provide the long-sought mechanism to heat and to extend the atmosphere of the star, and help to initiate the mass flow from the supergiant. In addition our monitoring program demonstrates that the longer 5.8 year period of variability identified in the 1930s has disappeared. A longer period has developed. We propose to continue the multifrequency monitoring of Alpha Ori for the next two years to determine the long period of variability, to establish the phase delay between chromospheric and photospheric modulation, and to evaluate the stability of the 420-day period. These observations represent a unique use of the IUE satellite since they provide continuous time line for a single object, enabling long-term periodicities to be discovered and allowing study of the response of a supergiant atmosphere and circumstellar shell to photospheric pulsations.
- Buscher 1990: High-resolution images of the M supergiant Betelgeuse in February 1989 at wavelengths of 633, 700, and 710 nm were made using the nonredundant masking method. At all these wavelengths, there is unambiguous evidence for an asymmetric feature on the surface of the star, which contributes 10-15 percent of the total observed flux. This might be due to a close companion passing in front of the stellar disk or, more likely, to large-scale convection in the stellar atmosphere.
- Dupree 1992: Our discovery of a 420-day periodic variation in the radiations from Alpha Orionis (Betelgeuse) was made possible by dedicated frequent observations with the IUE satellite in conjunction with contemporaneous ground-based photometric and spectroscopic observations. Periodic photospheric pulsations are the most likely explanation of the photometric variations, and this IUE result has recently been confirmed by measured velocity variations in the photosphere. Identification of pulsations in the atmosphere of Alpha Ori is important for they appear to be the fundamental pulsation mode of the star, and can provide the long-sought mechanism to heat and to extend the atmosphere of the star, and help to initiate the mass flow from the supergiant. Our monitoring program has demonstrated that the longer 5.8 year period of variability identified in the 1930s has disappeared, and a period of ~10.2 years has developed. We propose to continue the multifrequency monitoring of Alpha Ori for the next three years to determine the long period of variability, to establish the phase delay between chromospheric and photospheric modulation, and to evaluate the stability of the 420-day period. These obsservations represent a unique use of IUE since they provide continuous time line, enabling long-term periodicities to be discovered and allowing study of the response of a supergiant atmosphere and circumstellar shell to photospheric pulsations.
- Wilson 1992: Diffraction-limited images have been made of the M giant stars Alpha Orionis (Betelgeuse) and Omicron Ceti (Mira), using the nonredundant masking method at the 4.2-m William Herschel Telescope, for wavelengths between 546 and 710 nm. The brightness distribution for the surface of Betelgeuse is nonuniform, and has shown large changes over a period of 22 m. Karovska et al. (1986) reported two possible stellar companions to Alpha Ori. our maps show no features outside the stellar disc at the 2 sigma level, and we can set an upper limit on the brightness of any companion within 0.5 arcsec of the primary star as approximately 3.5 mag less than Betelgeuse, for wavelengths in the range 546 to 710 nm. The features we have detected on the surface of the star appear to be too bright to be associated with a passage of the suggested companion(s) in front of the red giant. They are also fainter at the 710 than at 700 nm, by a factor of 1.8, indicating that they lie within the moelcular atmosphere of the star.
- Karovska 1992: Alpha Ori is a cool supergiant star characterized by pronounced variability in most of its observed parameters. For more than a century it has been extensively studied by a variety of techniques, including speckle interferometry. The growing interest in this star during recent years is due in large part to the detection of a bright source in the vicinity of the star (possibly of a stellar nature).
- Karovska 2006: Interferometric Surveys and Results on Variabl Stars: Now and in the Future.
- Gilliland Dupree 1996:
Existing Refs
[edit]- AAVSO [28]
- BURNHAM [29]
- 100GREATEST [57]
- MICHELSON [58]
- BUSCHER [61]
- WILSON [81]
- HARPER [69]
- KALER [27]
- UC BERKELEY [7]
- ESCIENCE [66]
- SCHWARZSCHILD 1975 [33]
- FREYTAG 2002 [78]
- Kervella 2009 [35]
Notes
[edit]- ^ a b The calculations below illustrate the theoretical calculations for a higher luminosity assuming a radius of 5.5AU and an average temperature of 3,641K as follows: Cite error: The named reference "NOTELUMINOSITY" was defined multiple times with different content (see the help page).
- ^ a b c The following table provides a non-exhaustive list of angular measurements conducted since 1920. Also included is a column providing a current range of radii for each study based on α Ori's most recent distance estimate (Harper et al) of 197±45 pc:
Article Year1 Telescope # Spectrum λ (μm) ∅ (mas)2 Radii3 @
197±45 pcNotes Michelson 1920 Mt-Wilson 1 Visible 0.575 47.0 ± 4.7 3.2 - 6.3 AU Limb darkened +17% = 55.0 Bonneau 1972 Palomar 8 Visible 0.422-0.719 52.0 - 69.0 3.6 - 9.2 AU Strong correlation of ∅ with λ Balega 1978 ESO 3 Visible 0.405-0.715 45.0 - 67.0 3.1 - 8.6 AU No correlation of ∅ with λ 1979 SAO 4 Visible 0.575-0.773 50.0 - 62.0 3.5 - 8.0 AU Buscher 1989 WHT 4 Visible 0.633-0.710 54.0 - 61.0 4.0 - 7.9 AU Discovered asymmetries/hotspots Wilson 1991 WHT 4 Visible 0.546-0.710 49.0 - 57.0 3.5 - 7.1 AU Confirmation of hotspots Tuthill 1993 WHT 8 Visible 0.633-0.710 43.5 - 54.2 3.2 - 7.0 AU Study of hotspots on 3 stars 1992 WHT 1 NIR 0.902 42.6 ± 0:03 3.0 - 5.6 AU Weiner 1999 ISI 2 MIR (N Band) 11.150 54.7 ± 0.3 4.1 - 6.7 AU Limb darkened = 55.2 ± 0.5 Perrin 1997 IOTA 7 NIR (K Band) 2.200 43:33 ± 0:04 3.3 - 5.2 AU K&L Band,11.5μm data contrast Haubois 2005 IOTA 6 NIR (H Band) 1.650 44.28 ± 0.15‡ 3.4 - 5.4 AU Rosseland diameter 45.03 ± 0.12 Hernandez 2006 VLTI 2 NIR (K Band) 2.099-2.198 42:57 ± 0:02 3.2 - 5.2 AU High precision AMBER results. Ohnaka 2008 VLTI 3 NIR (K Band) 2.280-2.310 43.19 ± 0.03 3.3 - 5.2 AU Limb darkened 43.56 ± 0.06 Townes 1993 ISI 17 MIR (N Band) 11.150 56.00 ± 1.00 4.2 - 6.8 AU Systematic study involving 17 measurements at the same wavelength from 1993-2009 2008 ISI MIR (N Band) 11.150 47.00 ± 2.00 3.6 - 5.7 AU 2009 ISI MIR (N Band) 11.150 48.00 ± 1.00 3.6 - 5.8 AU Harper 2004 VLA Also noteworthy, Harper et al in the conclusion of their paper make the following remark: "In a sense, the derived distance of 200 pc is a balance between the 131 pc (425 ly) Hipparcos distance and the radio which tends towards 250 pc (815 ly)"—hence establishing ± 815 ly as the outside distance for the star. 1The final year of observations, unless otherwise noted. 2Uniform disk measurement, unless otherwise noted. 3Radii calculations use the same methodology as outlined in Note #2 below ‡Limb darkened measurement.
- ^ a b c Note coding Cite error: The named reference "NOTERADIUS" was defined multiple times with different content (see the help page).
- ^ a b Betelgeuse's radius at 56.0 mas is 5.516 AU (1,185 ), assuming a distance of 197.0 pc. Its radius at 47.0 mas would be 4.630 AU (995 ), hence a shrikange of 0.887 AU, a little more than the orbit of Venus, and just under the orbit of the Earth.
- ^ The analogy is based on the computation of certain ratios — specifically the diameter, radius and volume of the 3 celestial bodies in question, Betelgeuse, the Sun and Earth. Once these ratios are derived, the relative size of each as they relate to Wembley Stadium can be easily determined. The calculations begin with the formula for angular diameter as follows:
- Betelgeuse diameter ≈ 0.0552 arcseconds × 197.0 pc ≈ 11.000 AU (rounded up) × 149,597,871 km ≈ 1.646 ×109 km,
- Betelgeuse radius ≈ 11.000 AU ÷ 2 ≈ 5.500 AU × 149,597,871 km ≈ 8.230 ×108 km ≈ 823,000,000 km,
- Betelgeuse volume ≈ (4÷3×π) × 823,000,0003 ≈ 2.335 × 1027 km3.
- Solar radius ≈ 696,000 km. Volume ≈ 1.412×1018 km3,
- Earth radius ≈ 6,371.0 km. Volume ≈ 1.083×1012 km3.
- Wembley Bowl Volume ≈ 1,139,100 m3. Spherical radius ≈ (1,139,100m3 ÷ (4/3*π))1/3 ≈ 64.787 m or 64,787 mm.
- Betelgeuse ≈ (2.335 × 1027) ÷ (1.412×1018) ≈ 1.654 × 109 Suns,
- Betelgeuse ≈ (2.335 × 1027) ÷ (1.083×1012) ≈ 2.156 × 1015 Earths.
- Solar volume relative to Wembley ≈ 1,139,100 m3 ÷ (1.654 × 109) × 109{i.e. to convert to mm3} ≈ 689,000 mm3 (rounded up)
- Solar diameter relative to Wembley ≈ (689,000 mm3 ÷ (4/3*π))1/3 ≈ 55.61845 × 2 ≈ 110 mm or 4.3 inches
- Earth volume relative to Wembley ≈ 1,139,100 m3 ÷ (2.156 × 1015) × 109{i.e. to convert to mm3} ≈ 0.528 mm3
- Earth diameter relative to Wembley ≈ (0.528 mm3 ÷ (4/3*π))1/3 ≈ 0.501 × 2 ≈ 1.002 mm or 0.04 inches
- Earth's orbital radius (1 AU) relative to Wembley ≈ 1AU ÷ 5.5 AU × 64.787 meters = 11.8 meters.
- If the immense space of Wembley Stadium were Betelgeuse, the Earth would be a tiny pearl, 1.0 mm in diameter, orbiting a Sun, 11.0 cm/4.3 inches in diameter (i.e. the size of an average mango or grapefruit), with an orbital distance of about 11.8 meters.
- ^ Once again, the calculations begin with the formula for angular diameter as follows:
Calculations for 1993 values:
- Betelgeuse radius ≈ 0.056 arcseconds × 197.0 pc ≈ 11.032 AU ÷ 2 ≈ 5.516 AU × 149,597,871 km ≈ 825,000,000 km,
- Betelgeuse volume ≈ (4÷3×π) × 825,000,0003 ≈ 2.352 × 1027 km3.
- Betelgeuse radius ≈ 0.047 arcseconds × 197.0 pc ≈ 9.260 AU ÷ 2 ≈ 4.630 AU × 149,597,871 km ≈ 692,500,000 km,
- Betelgeuse volume ≈ (4÷3×π) × 692,500,0003 ≈ 1.391 × 1027 km3.
- Betelgeuse change in volume ≈ 2.352 × 1027 km3 – 1.391 × 1027 km3 ≈ –9.610E × 1026 km3
- Betelgeuse percent change in volume ≈ –9.610E × 1026 km3 ÷ 2.352 × 1027 km3 ≈ –40.86%
- Betelgeuse volume change as a function of Solar volume ≈ –9.610E × 1026 ÷ 1.412×1018 km3 ≈ –681,000,000 Suns.
- ^ To compute these density ratios, the first step is to calculate the mass and volume of each component from which density calculations can be made. Once we have the density of the Sun, Betelgeuse, and the Earth's atmosphere, then it's simply a matter of simple division to compute the appropriate ratios. Therefore:
Calculations for the Sun:- Solar mass ≈ 1.9891×1030 kg
- Solar volume ≈ 1.412×1018 km3.
- Solar density ≈ 1.9891×1030 ÷ (1.412×1018 × 109) ≈ 1.409×103 kg/m3.
- Betelgeuse mass ≈ 18.5 x Solar × 1.9891×1030 ≈ 3.680 ×1031 kg
- Betelgeuse volume ≈ 2.335 × 1027 km3 (from previous note)
- Betelgeuse density ≈ 3.680 ×1031 kg ÷ 2.335 × 1027 km3 × 109) ≈ 1.576 ×10-5 kg/m3.
- Atmospheric Mass ≈ 5.148 × 1018 kg
- Atmospheric Volume ≈ 4.200 × 1009 km3.
- Atmospheric Density ≈ 5.148 × 1018 kg ÷ 4.200 × 1009 km3 × 109) ≈ 1.226 kg/m3.
- Betelgeuse/Sun ≈ 1.576 × 10-5 kg/m3 ÷ 1.409 × 103 kg/m3 ≈ 1.116 × 10-23 kg/m3
- Betelgeuse/Earth's Atmosphere = 1.576 × 10-5 kg/m3 ÷ 1.226 × 100 kg/m3 ≈ 1.286 × 10-5 kg/m3 OR 1.286 × 10-8 g/cm3
- Betelgeuse's average density relative to Earth's Atmosphere is graphically represented in the NRLMSISE-00 standard atmosphere model. A density of 1.286 × 10-8 g/cm3, is roughly equivalent to the Earth's atmospheric density found at about 90 kilometers above sea level. Noctilucent clouds are found between 76 to 85 km above sea level.
References
[edit]- ^ van Leeuwen, F (November 2007). "Hipparcos, the New Reduction". Astronomy and Astrophysics. 474 (2): 653–664. arXiv:0708.1752. Bibcode:2007A&A...474..653V. doi:10.1051/0004-6361:20078357. S2CID 18759600.
{{cite journal}}
: Unknown parameter|duplicate-journal=
ignored (help)CS1 maint: date and year (link) - ^ For detailed computations of stellar radius and implications relating to the star's luminosity, see the Computational challenges section in the Luminosity article
- ^ a b c d e Weiner, J.; Danchi, W. C.; Hale, D. D. S.; McMahon, J.; et al. (2000). "Precision Measurements of the Diameters of α Orionis and ο Ceti at 11 Microns" (PDF). The Astrophysical Journal. 544 (2): 1097–1100. Bibcode:2000ApJ...544.1097W. doi:10.1086/317264. S2CID 11697011. Retrieved 2007-06-23.
{{cite journal}}
: Explicit use of et al. in:|author=
(help)CS1 maint: multiple names: authors list (link) Cite error: The named reference "WEINER" was defined multiple times with different content (see the help page). - ^ Smith, Nathan; Hinkle, Kenneth H.; Ryde, Nils (March 2009). "Red Supergiants as Potential Type IIn Supernova Progenitors: Spatially Resolved 4.6 μm CO Emission Around VY CMa and Betelgeuse" (PDF). The Astronomical Journal. 137 (3): 3558–3573. arXiv:0811.3037. Bibcode:2009AJ....137.3558S. doi:10.1088/0004-6256/137/3/3558. S2CID 19019913. Retrieved 9 September 2012.
{{cite journal}}
: CS1 maint: date and year (link) CS1 maint: multiple names: authors list (link) - ^ a b Robert Nemiroff (MTU) & Jerry Bonnell (USRA) (6 January 2010). "The Spotty Surface of Betelgeuse". Today's Astronomy Picture of the Day. Retrieved 18 July 2010. Cite error: The named reference "APOD2" was defined multiple times with different content (see the help page).
- ^ a b c d e f g h Perrin, G.; Ridgway, S. T.; Coudé du Foresto, V.; Mennesson, B.; Traub, W. A.; Lacasse, M. G. (May 2004). "Interferometric observations of the supergiant stars α Orionis and α Herculis with FLUOR at IOTA". Astronomy and Astrophysics. 418 (2): 675–85. arXiv:astro-ph/0402099. Bibcode:2004A&A...418..675P. doi:10.1051/0004-6361:20040052. S2CID 18648216. Retrieved 2007-08-06.
{{cite journal}}
: Unknown parameter|origin=
ignored (help)CS1 maint: date and year (link) CS1 maint: multiple names: authors list (link) - ^ a b c d e f g Sanders, Robert (2009). "Red giant star Betelgeuse mysteriously shrinking". UC Berkeley News. UC Berkeley. Retrieved 18 April 2010.
The measurements cannot be compared anyway, because the star's size depends on the wavelength of light used to measure it, Townes said. This is because the tenuous gas in the outer regions of the star emits light as well as absorbs it, which makes it difficult to determine the edge of the star.
{{cite web}}
: Unknown parameter|month=
ignored (help) Cite error: The named reference "UC BERKELEY" was defined multiple times with different content (see the help page). - ^ NASA/JPL-Caltech/UCLA (Last update: 18 April 2011). "Orion's Big Head Revealed in Infrared". NASA Mission News. Retrieved 12 June 2012.
{{cite web}}
: Check date values in:|date=
(help) - ^ a b c d Accurate diameter measurement of Betelgeuse using the VLTI/AMBER instrument; Chelli (November 2009). "Hernandez Utrera, O.; Chelli, A" (PDF). Revista Mexicana de Astronomía y Astrofísica (Serie de Conferencias). 37: 179–80. Bibcode:2009RMxAC..37..179H.
{{cite journal}}
: CS1 maint: date and year (link) Cite error: The named reference "HERNANDEZ" was defined multiple times with different content (see the help page). - ^ a b c d e f g h i j k l m n o Haubois, X.; Perrin, G.; Lacour, S.; Verhoelst, T.; Meimon, S.; et al. (December 2009). "Imaging the spotty surface of Betelgeuse in the H band". Astronomy and Astrophysics. 508 (2): 923–32. arXiv:0910.4167. Bibcode:2009A&A...508..923H. doi:10.1051/0004-6361/200912927. S2CID 118593802.
{{cite journal}}
: CS1 maint: date and year (link) Cite error: The named reference "HAUBOIS" was defined multiple times with different content (see the help page). - ^ Ohnaka, K.; Weigelt, G.; Millour, F.; Hofmann, K.-H.; Driebe, T.; Schertl, D.; Chelli, A.; Massi, F.; Petrov, R.; Stee, Ph. (May 2011). "Imaging the dynamical atmosphere of the red supergiant Betelgeuse in the CO first overtone lines with VLTI/AMBER" (PDF). Astronomy & Astrophysics, Volume 529, Id.A163. 529, id.A163: A163. arXiv:1104.0958. Bibcode:2011A&A...529A.163O. doi:10.1051/0004-6361/201016279. S2CID 56281923. Retrieved 14 September 2012.
We derive a uniform-disk diameter of 42.05 ± 0.05 mas and a power-law-type limb-darkened disk diameter of 42.49 ± 0.06 mas and a limb-darkening parameter of (9.7 ± 0.5) × 10-2
{{cite journal}}
: CS1 maint: date and year (link) CS1 maint: multiple names: authors list (link) - ^ Kervella, P.; Perrin, G.; Chiavassa, A.; Ridgway, S. T.; Cami, J.; Haubois, X.; Verhoelst, T. (July 2011). "The close circumstellar environment of Betelgeuse. II. Diffraction-limited spectro-imaging from 7.76 to 19.50 μm with VLT/VISIR" (PDF). Astronomy & Astrophysics, Volume 531, Id.A117. 531, id.A117: A117. arXiv:1106.5041. Bibcode:2011A&A...531A.117K. doi:10.1051/0004-6361/201116962. S2CID 119190969. Retrieved 14 September 2012.
{{cite journal}}
: CS1 maint: date and year (link) CS1 maint: multiple names: authors list (link) - ^ a b c d e Townes, C. H.; Wishnow, E. H.; Hale, D. D. S.; Walp, B. (June 2009). "A Systematic Change with Time in the Size of Betelgeuse". The Astrophysical Journal Letters. 697 (2): L127-28. Bibcode:2009ApJ...697L.127T. doi:10.1088/0004-637X/697/2/L127. S2CID 121009337. Retrieved 2007-08-08.
{{cite journal}}
: Unknown parameter|origin=
ignored (help)CS1 maint: date and year (link) CS1 maint: multiple names: authors list (link) - ^ a b Cowen, Ron (10 June 2009). "Betelgeuse shrinks: The red supergiant has lost 15 percent of its size".
The shrinkage corresponds to the star contracting by a distance equal to that between Venus and the Sun, researchers reported June 9 at an American Astronomical Society meeting and in the June 1 Astrophysical Journal Letters.
Cite error: The named reference "COWEN" was defined multiple times with different content (see the help page). - ^ Courtland, Rachel (2009). "Betelgeuse: The incredible shrinking star?". New Scientist. Reed Business Information Ltd. Retrieved 25 September 2010.
{{cite web}}
: Unknown parameter|month=
ignored (help) - ^ Cite error: The named reference
RAVI1
was invoked but never defined (see the help page). - ^ a b Bedding, T. R.; Zijlstra, A. A.; Von Der Luhe, O.; Robertson, J. G.; et al. (1997). "The angular diameter of R Doradus: a nearby Mira-like star". Monthly Notices of the Royal Astronomical Society. 286 (4): 957–62. arXiv:astro-ph/9701021. Bibcode:1997MNRAS.286..957B. doi:10.1093/mnras/286.4.957.
{{cite journal}}
: CS1 maint: unflagged free DOI (link) Cite error: The named reference "BEDDING" was defined multiple times with different content (see the help page). - ^ a b Karovska, M.; Noyes, R. W.; Roddier, F.; Nisenson, P.; Stachnik, R. V.; Noyes; Roddier; Nisenson; Stachnik (1985). "On a Possible Close Companion to α Ori". Bulletin of the American Astronomical Society. 17: 598. Bibcode:1985BAAS...17..598K.
{{cite journal}}
: CS1 maint: multiple names: authors list (link) Cite error: The named reference "KAROVSKA1" was defined multiple times with different content (see the help page). - ^ a b Karovska, M.; Nisenson, P.; Noyes, R. (1986). "On the alpha Orionis triple system". Astrophysical Journal. 308: 675–85. Bibcode:1986ApJ...308..260K. doi:10.1086/164497.
{{cite journal}}
: CS1 maint: multiple names: authors list (link) Cite error: The named reference "KAROVSKA2" was defined multiple times with different content (see the help page). - ^ a b Wilson, R. W.; Baldwin, J. E.; Buscher, D. F.; Warner, P. J.; Baldwin; Buscher; Warner (1992). "High-resolution imaging of Betelgeuse and Mira". Monthly Notices of the Royal Astronomical Society. 257 (3): 369–76. Bibcode:1992MNRAS.257..369W. doi:10.1093/mnras/257.3.369.
{{cite journal}}
: CS1 maint: multiple names: authors list (link) CS1 maint: unflagged free DOI (link) Cite error: The named reference "WILSON2" was defined multiple times with different content (see the help page). - ^ a b Karovska, M. (1992). "Imaging of the Surface of α ORI". Proceedings of the 7th Cambridge Workshop, ASP Conference Series. 26: 279. Bibcode:1992ASPC...26..279K. Cite error: The named reference "KAROVSKA3" was defined multiple times with different content (see the help page).
- ^ "CCDM (Catalog of Components of Double & Multiple stars (Dommanget+ 2002)". VizieR. Centre de Données astronomiques de Strasbourg. Retrieved 22 August 2010.
- ^ "ESA space operations and situational awareness". European Space Agency. Last update: 22 April 2010. Retrieved 15 November 2010.
{{cite web}}
: Check date values in:|date=
(help) - ^ Neilson, H. R.; Lester, J. B.; Haubois, X. (December 2011). "Weighing Betelgeuse: Measuring the Mass of α Orionis from Stellar Limb-darkening" (PDF). Astronomical Society of the Pacific. 9th Pacific Rim Conference on Stellar Astrophysics. Proceedings of a conference held at Lijiang, China in 14–20 April 2011. ASP Conference Series, Vol. 451: 117. arXiv:1109.4562. Bibcode:2010ASPC..425..103L. Retrieved 9 September 2012.
{{cite journal}}
: CS1 maint: date and year (link) CS1 maint: multiple names: authors list (link) - ^ a b c d Posson-Brown, Jennifer; Kashyap, Vinay L.; Pease, Deron O.; Drake, Jeremy J. (2006). "Dark Supergiant: Chandra's Limits on X-rays from Betelgeuse". arXiv:astro-ph/0606387.
{{cite arXiv}}
: CS1 maint: multiple names: authors list (link) - ^ Gray, David F. (March 2000). "Betelgeuse and Its Variations" (PDF). The Astrophysical Journal. 532 (1): 487–96. Bibcode:2000ApJ...532..487G. doi:10.1086/308538. S2CID 121170944.
The mass of the star is unknown, but most investigators show a preference for a fairly large mass in the range of 10–20M☉
{{cite journal}}
: CS1 maint: date and year (link) - ^ a b c d e Kaler, James B. (Jim). "Betelgeuse (Alpha Orionis)". Stars website. University of Illinois. Retrieved 19 July 2009. Cite error: The named reference "KALER" was defined multiple times with different content (see the help page).
- ^ a b c d e f g h i j k l Davis, Kate (AAVSO Technical Assistant, Web) (December 2000). "Variable Star of the Month: Alpha Orionis". American Association of Variable Star Observers (AAVSO). Retrieved 2010-07-10.
{{cite web}}
: CS1 maint: date and year (link) CS1 maint: multiple names: authors list (link) Cite error: The named reference "AAVSO" was defined multiple times with different content (see the help page). - ^ a b c d e Burnham, Robert (1978). Burnham's Celestial Handbook: An Observer's Guide to the Universe Beyond the Solar System, Volume 2. New York: Courier Dover Publications. p. 1290. ISBN 0486235688.
- ^ Schiller, F.; Przybilla, N. (March 2008). "Quantitative spectroscopy of Deneb" (PDF). Astronomy & Astrophysics. 479 (3): 849–858. arXiv:0712.0040v1. Bibcode:2008A&A...479..849S. doi:10.1051/0004-6361:20078590. S2CID 103635615. Retrieved 30 June 2012.
Earlier data had yielded a luminosity of 54,000L☉ with a radius of 108R☉
{{cite journal}}
: CS1 maint: date and year (link) CS1 maint: multiple names: authors list (link) - ^ a b c d e f g "Sharpest views of Betelgeuse reveal how supergiant stars lose mass". Press Releases. European Southern Observatory. 2009. Retrieved 2010-09-06.
The image is reproduced in the Properties section below.
{{cite web}}
: Unknown parameter|month=
ignored (help) - ^ a b
Ridgway, Stephen; Aufdenberg, Jason; Creech-Eakman, Michelle; Elias, Nicholas; et al. (2009). "Quantifying Stellar Mass Loss with High Angular Resolution Imaging". Astronomy and Astrophysics. 247: 247. arXiv:0902.3008. Bibcode:2009astro2010S.247R.
Ridgway notes: 'Stellar mass loss is key to understanding the evolution of the universe from the earliest cosmological times to the current epoch, and of planet formation and the formation of life itself.'
- ^ a b c d e f g h Schwarzschild, Martin (1975). "On the scale of photospheric convection in red giants and supergiants" (PDF). Astrophysical Journal. 195, pt. 1: 137–144. Bibcode:1975ApJ...195..137S. doi:10.1086/153313. Retrieved 2010-07-26.
{{cite journal}}
: Unknown parameter|month=
ignored (help); Unknown parameter|origin=
ignored (help) Cite error: The named reference "SCHWARZSCHILD1975" was defined multiple times with different content (see the help page). - ^ a b c Gilliland, Ronald L.; Dupree, Andrea K. (May 1996). "First Image of the Surface of a Star with the Hubble Space Telescope" (PDF). Astrophysical Journal Letters. 463: L29. Bibcode:1996ApJ...463L..29G. doi:10.1086/310043. S2CID 117717866. Retrieved 2010-08-01.
{{cite journal}}
: Unknown parameter|origin=
ignored (help)CS1 maint: date and year (link) CS1 maint: multiple names: authors list (link) - ^ a b c d e f Kervella, P.; Verhoelst, T.; Ridgway, S. T.; Perrin, G.; et al. (2009, September). "The close circumstellar environment of Betelgeuse. Adaptive optics spectro-imaging in the near-IR with VLT/NACO". Astronomy and Astrophysics. 504 (1): 115–25. arXiv:0907.1843. Bibcode:2009A&A...504..115K. doi:10.1051/0004-6361/200912521. S2CID 14278046. Retrieved 2010-07-10.
{{cite journal}}
: Check date values in:|date=
(help); Explicit use of et al. in:|author=
(help); Unknown parameter|origin=
ignored (help)CS1 maint: multiple names: authors list (link) - ^ a b A. P.Ohnaka, K.; Hofmann, K.-H.; Benisty, M.; Chelli, A.; et al. (2009). "Spatially resolving the inhomogeneous structure of the dynamical atmosphere of Betelgeuse with VLTI/AMBER". Astronomy and Astrophysics. 503 (1): 183–95. arXiv:0906.4792. Bibcode:2009A&A...503..183O. doi:10.1051/0004-6361/200912247. S2CID 17850433.
- ^ a b Tsuji, T. (2000). "Water on the Early M Supergiant Stars α Orionis and μ Cephei" (PDF). The Astrophysical Journal. 538 (2): 801–07. Bibcode:2000ApJ...538..801T. doi:10.1086/309185. S2CID 122207991.
- ^ a b Lambert, D. L.; Brown, J. A.; Hinkle, K. H.; Johnson, H. R. (September 1984). "Carbon, nitrogen, and oxygen abundances in Betelgeuse". Astrophysical Journal. 284: 223–37. Bibcode:1984ApJ...284..223L. doi:10.1086/162401.
{{cite journal}}
: CS1 maint: date and year (link) CS1 maint: multiple names: authors list (link) - ^ a b c d e f g Dave Finley (April 8, 1998). "VLA Shows "Boiling" in Atmosphere of Betelgeuse". National Radio Astronomy Observatory. Retrieved 7 September 2010. Cite error: The named reference "NRAO" was defined multiple times with different content (see the help page).
- ^ a b
Lim, Jeremy; Carilli, Chris L.; White, Stephen M.; Beasley, Anthony J.; Marson, Ralph G. (1998). "Large convection cells as the source of Betelgeuse's extended atmosphere". Nature. 392 (6676): 575–77. Bibcode:1998Natur.392..575L. doi:10.1038/33352. S2CID 4431516.
{{cite journal}}
: CS1 maint: multiple names: authors list (link) - ^ a b Cite error: The named reference
DUPREE
was invoked but never defined (see the help page). - ^ a b c d
Lobel, A.; Aufdenberg, J.; Dupree, A. K.; Kurucz, R. L.; Stefanik, R. P.; Torres, G.; Aufdenberg; Dupree; Kurucz; Stefanik; Torres (2004). "Spatially Resolved STIS Spectroscopy of Betelgeuse's Outer Atmosphere". Publications of the Astronomical Society of the Pacific. 219: 641. arXiv:astro-ph/0312076. Bibcode:2004IAUS..219..641L.
In the article, Lobel et al. equate 1 arcsecond to approximately 40 stellar radii, a calculation which in 2004 likely assumed a Hipparcos distance of 131 pc (430 ly) and a photospheric diameter of 0.0552" from Weiner et al.
{{cite journal}}
: CS1 maint: multiple names: authors list (link) - ^ a b "The Flames of Betelgeuse". ESO Photo Release. 23 June 2011. Retrieved 24 June 2011.
- ^ a b Robert Nemiroff (MTU) & Jerry Bonnell (USRA) (28 June 2011). "Stardust and Betelgeuse". Today's Astronomy Picture of the Day. Retrieved 10 June 2012.
- ^ a b
Sutton, E. C.; Storey, J. W. V.; Betz, A. L.; Townes, C. H.; Spears, D. L. (1977). "Spatial heterodyne interferometry of VY Canis Majoris, Alpha Orionis, Alpha Scorpii, and R Leonis at 11 microns". Astrophysical Journal Letters. 217: L97–L100. Bibcode:1977ApJ...217L..97S. doi:10.1086/182547.
{{cite journal}}
: CS1 maint: multiple names: authors list (link) - ^ a b c d
Skinner, C. J.; Dougherty, S. M.; Meixner, M.; Bode, M. F.; Davis, R. J.; et al. (1997). "Circumstellar environments – V. The asymmetric chromosphere and dust shell of alpha Orionis". Monthly Notices of the Royal Astronomical Society. 288 (2): 295–306. Bibcode:1997MNRAS.288..295S. doi:10.1093/mnras/288.2.295.
{{cite journal}}
: CS1 maint: unflagged free DOI (link) - ^ a b
Danchi, W. C.; Bester, M.; Degiacomi, C. G.; Greenhill, L. J.; Townes, C. H. (1994). "Characteristics of dust shells around 13 late-type stars". The Astronomical Journal. 107 (4): 1469–1513. Bibcode:1994AJ....107.1469D. doi:10.1086/116960.
{{cite journal}}
: CS1 maint: multiple names: authors list (link) - ^ a b
Baud, B.; Waters, R.; De Vries, J.; Van Albada, G. D.; et al. (January 1984). "A Giant Asymmetric Dust Shell around Betelgeuse". Bulletin of the American Astronomical Society. 16: 405. Bibcode:1984BAAS...16..405B.
{{cite journal}}
: CS1 maint: date and year (link) - ^ a b
David, L.; Dooling, D.; Dooling (February 1984). "The infrared universe". Space World. 2: 4–7. Bibcode:1984SpWd....2....4D.
{{cite journal}}
: CS1 maint: date and year (link) CS1 maint: multiple names: authors list (link) - ^ a b Harper, Graham M.; Carpenter, Kenneth G.; Ryde, Nils; Smith, Nathan; Brown, Joanna; et al. (2009). "UV, IR, and mm Studies of CO Surrounding the Red Supergiant α Orionis (M2 Iab)". AIP Conference Proceedings. 1094: 868–871. Bibcode:2009AIPC.1094..868H. doi:10.1063/1.3099254.
- ^ a b "Akari infrared space telescope: latest science highlights". European Space Agency. 19 November 2008. Retrieved 25 June 2012.
- ^ a b Noriega-Crespo, Alberto; van Buren, Dave; Cao, Yu; Dgani, Ruth (August 1997). "A Parsec-Size Bow Shock around Betelgeuse" (PDF). Astronomical Journal. 114: 837–40. Bibcode:1997AJ....114..837N. doi:10.1086/118517. Retrieved 25 June 2012.
Noriega in 1997 estimated the size to be 0.8 parsecs, having assumed the earlier distance estimate of 400ly. With a current distance estimate of 643ly, the bow shock would measure ~1.28 parsecs or over 4 ly
{{cite journal}}
: CS1 maint: date and year (link) CS1 maint: multiple names: authors list (link) - ^ a b Elizabeth Newton (26 April 2012). "This star lives in exciting times, or, How did Betelgeuse make that funny shape?". Astrobites. Retrieved 2012-06-25.
- ^ a b Mohamed, S.; Mackey, J.; Langer, N. (May 2012). "3D simulations of Betelgeuse's bow shock". Astronomy & Astrophysics. 541, id.A1: A1. arXiv:1109.1555. Bibcode:2012A&A...541A...1M. doi:10.1051/0004-6361/201118002. S2CID 118435586.
{{cite journal}}
: CS1 maint: date and year (link) CS1 maint: multiple names: authors list (link) - ^ a b Mackey, Jonathan; Mohamed, Shazrene; Neilson, Hilding R.; Langer, Norbert; Meyer, Dominique M.-A.; et al. (May 2012). "Double Bow Shocks around Young, Runaway Red Supergiants: Application to Betelgeuse". The Astrophysical Journal Letters. 751 (1, article id. L10): 837–40. arXiv:1204.3925. Bibcode:2012ApJ...751L..10M. doi:10.1088/2041-8205/751/1/L10. S2CID 118433862.
{{cite journal}}
: CS1 maint: date and year (link) - ^ Cite error: The named reference
allen
was invoked but never defined (see the help page). - ^ a b c Kaler, James B. (2002). The Hundred Greatest Stars. New York: Copernicus Books. p. 33. ISBN 0-387-95436-8.
- ^ a b c d e Michelson, Albert Abraham; Pease, Francis G. (1921). "Measurement of the diameter of alpha Orionis with the interferometer" (PDF). Astrophysical Journal. 53: 249–59. Bibcode:1921ApJ....53..249M. doi:10.1086/142603. S2CID 21969744. Retrieved 2007-06-20.
{{cite journal}}
: CS1 maint: multiple names: authors list (link) Cite error: The named reference "MICHELSON" was defined multiple times with different content (see the help page). - ^ Schwarzschild, Martin (1958). Structure and evolution of the stars. Princeton University Press. Bibcode:1958ses..book.....S.
- ^ The yellow/red "image" or "photo" of Betelgeuse usually seen is actually not a picture of the red giant but rather a mathematically generated image based on the photograph. The photograph was actually of much lower resolution: The entire Betelgeuse image fit entirely within a 10x10 pixel area on the Hubble Space Telescopes Faint Object Camera. The actual images were oversampled by a factor of 5 with bicubic spline interpolation, then deconvolved.
- ^ a b c d e Buscher, D. F.; Baldwin, J. E.; Warner, P. J.; Haniff, C. A. (1990). "Detection of a bright feature on the surface of Betelgeuse" (PDF). Monthly Notices of the Royal Astronomical Society. 245: 7. Bibcode:1990MNRAS.245P...7B. Retrieved 2007-08-07.
{{cite journal}}
: CS1 maint: multiple names: authors list (link) Cite error: The named reference "BUSCHER" was defined multiple times with different content (see the help page). - ^ Wilson, R. W.; Dhillon, V. S.; Haniff, C. A. (1997). "The changing face of Betelgeuse" (PDF). Monthly Notices of the Royal Astronomical Society. 291 (4): 819. Bibcode:1997MNRAS.291..819W. doi:10.1093/mnras/291.4.819. Retrieved 2007-08-07.
{{cite journal}}
: CS1 maint: multiple names: authors list (link) CS1 maint: unflagged free DOI (link) - ^ Tuthill, P. G.; Haniff, C. A.; Baldwin, J. E. (March 1997). "Hotspots on late-type supergiants" (PDF). Monthly Notices of the Royal Astronomical Society. 285 (3): 529–539. Bibcode:1997MNRAS.285..529T. doi:10.1093/mnras/285.3.529. Retrieved 2010-09-07.
{{cite journal}}
: Unknown parameter|origin=
ignored (help)CS1 maint: date and year (link) CS1 maint: multiple names: authors list (link) CS1 maint: unflagged free DOI (link) - ^ Petersen, Carolyn Collins; Brandt, John C. (1998) [1995]. Hubble Vision: Further Adventures with the Hubble Space Telescope (2nd ed.). Cambridge, England: Cambridge University Press. pp. 91–92. ISBN 0-521-59291-7.
{{cite book}}
: CS1 maint: multiple names: authors list (link) - ^ Uitenbroek, Han; Dupree, Andrea K.; Gilliland, Ronald L. (1998). "Spatially Resolved Hubble Space Telescope Spectra of the Chromosphere of α Orionis". The Astronomical Journal. 116 (5): 2501–12. Bibcode:1998AJ....116.2501U. doi:10.1086/300596. S2CID 117596395. Retrieved 2007-06-20.
{{cite journal}}
: CS1 maint: multiple names: authors list (link) - ^ a b "Red giant star Betelgeuse is mysteriously shrinking". e! Science News. 2009. Retrieved 18 April 2010.
{{cite web}}
: Unknown parameter|month=
ignored (help) Cite error: The named reference "ESCIENCE" was defined multiple times with different content (see the help page). - ^ Townes, Charles H.; Wishnow, E. H.; Hale, D. D. S.; Walp, B. (2009). "A Systematic Change with Time in the Size of Betelgeuse". Astrophysical Journal Letters. 697 (2): L127. Bibcode:2009ApJ...697L.127T. doi:10.1088/0004-637X/697/2/L127. S2CID 121009337.
- ^ M. Aurière, J.-F. Donati, R. Konstantinova Antova, G. Perrin, P. Petit, T. Roudier (2010). "The magnetic field of Betelgeuse : a local dynamo from giant convection cells ?". Astronomy & Astrophysics. 516: L2. arXiv:1005.4845. doi:10.1051/0004-6361/201014925. S2CID 54943572.
{{cite journal}}
: CS1 maint: multiple names: authors list (link) - ^ a b c d e f g h i j Graham M. Harper, Alexander Brown, and Edward F. Guinan (2008, April). "A New VLA-Hipparcos Distance to Betelgeuse and its Implications" (PDF). The Astronomical Journal. 135 (4): 1430–1440. Bibcode:2008AJ....135.1430H. doi:10.1088/0004-6256/135/4/1430. S2CID 34660949. Retrieved 2010-07-10.
{{cite journal}}
: Check date values in:|date=
(help); Unknown parameter|origin=
ignored (help)CS1 maint: multiple names: authors list (link) - ^ van Altena, W. F.; Lee, J. T.; Hoffleit, D. (1995, October). "Yale Trigonometric Parallaxes Preliminary". Yale University Observatory (1991). Bibcode:1995yCat.1174....0V.
{{cite journal}}
: Check date values in:|date=
(help); Unknown parameter|origin=
ignored (help)CS1 maint: multiple names: authors list (link) - ^ "Hipparcos Input Catalogue, Version 2 (Turon+ 1993)". VizieR. Centre de Données astronomiques de Strasbourg. 1993. Retrieved 2010-06-20.
- ^ Perryman, M. A. C.; Lindegren, L.; Kovalevsky, J.; Hoeg, E.; et al. (1997). "The HIPPARCOS Catalogue" (PDF). Astronomy and Astrophysics. 323: L49–L52. Bibcode:1997A&A...323L..49P. Retrieved 2010-05-17.
{{cite journal}}
: Explicit use of et al. in:|author=
(help)CS1 maint: multiple names: authors list (link) - ^ Eyer, L.; Grenon, M. (2000). "Problems Encountered in the Hipparcos Variable Stars Analysis" (PDF). Delta Scuti and Related Stars, Reference Handbook and Proceedings of the 6th Vienna Workshop in Astrophysics. ASP Conference Series, Vol. 210: 482. arXiv:astro-ph/0002235. Bibcode:2000ASPC..210..482E. ISBN 1-58381-041-2. Retrieved 2010-07-14.
{{cite journal}}
: Unknown parameter|origin=
ignored (help)CS1 maint: multiple names: authors list (link) - ^ (Bessell 2000)
- ^ "Gaia overview". European Space Agency. Last update: 4 May 2010. Retrieved 2010-07-14.
{{cite web}}
: Check date values in:|date=
(help) - ^ a b c Goldberg, Leo (May 1984). "The variability of alpha Orionis" (PDF). Astronomical Society of the Pacific. 96. Publications (ISSN 0004-6280): 366–371. Bibcode:1984PASP...96..366G. doi:10.1086/131347. S2CID 121926262. Retrieved 2010-06-10.
{{cite journal}}
: Unknown parameter|origin=
ignored (help)CS1 maint: date and year (link) - ^ a b c d e f g h i j k l SolStation. "Betelgeuse". Sol Company. Retrieved 2010-07-20.
- ^ a b Freytag, B.; Steffen, M.; Dorch, B. (July 2002). "Spots on the surface of Betelgeuse -- Results from new 3D stellar convection models". Astronomische Nachrichten. 323 (3/4): 213–219. Bibcode:2002AN....323..213F. doi:10.1002/1521-3994(200208)323:3/4<213::AID-ASNA213>3.0.CO;2-H. Retrieved 2010-07-26.
{{cite journal}}
: Unknown parameter|origin=
ignored (help)CS1 maint: date and year (link) CS1 maint: multiple names: authors list (link) Cite error: The named reference "FREYTAG" was defined multiple times with different content (see the help page). - ^ LEIGHTON
- ^ Dupree, A. K.; Gilliland, R. L. (December 1995). "HST Direct Image of Betelgeuse". Bulletin of the American Astronomical Society. 27: 1328. Bibcode:1995AAS...187.3201D.
{{cite journal}}
: Unknown parameter|origin=
ignored (help)CS1 maint: date and year (link) CS1 maint: multiple names: authors list (link) - ^ a b Wilson, R. W.; Dhillon, V. S.; Haniff, C. A. (1997). "The changing face of Betelgeuse" (PDF). Monthly Notices of the Royal Astronomical Society. 291 (4): 819. Bibcode:1997MNRAS.291..819W. doi:10.1093/mnras/291.4.819. Retrieved 2007-08-07.
{{cite journal}}
: CS1 maint: multiple names: authors list (link) CS1 maint: unflagged free DOI (link) - ^ David Aguilar, Christine Pulliam, Dr. A. Lobel (2004). "Storms Of Hot And Cold Gas Rage In Betelgeuse's Turbulent Atmosphere". Harvard–Smithsonian Center for Astrophysics. Retrieved 2010-07-27.
{{cite web}}
: Unknown parameter|month=
ignored (help)CS1 maint: multiple names: authors list (link) - ^ a b Bonneau, D.; Labeyrie, A. (April 1973). "Speckle Interferometry: Color-Dependent Limb Darkening Evidenced on Alpha Orionis and Omicron Ceti" (PDF). Astrophysical Journal. 181: L1. Bibcode:1973ApJ...181L...1B. doi:10.1086/181171. Retrieved 2010-06-10.
{{cite journal}}
: Unknown parameter|origin=
ignored (help)CS1 maint: date and year (link) CS1 maint: multiple names: authors list (link) - ^ a b Balega, Iu.; Blazit, A.; Bonneau, D.; Koechlin, L.; Labeyrie, A.; Foy, R.. (Nov. 1982). "The angular diameter of Betelgeuse" (PDF). Astronomy and Astrophysics. 115 (2): 253–56. Bibcode:1982A&A...115..253B. Retrieved 2010-08-02.
{{cite journal}}
: Check date values in:|date=
(help); Unknown parameter|month=
ignored (help); Unknown parameter|origin=
ignored (help)CS1 maint: multiple names: authors list (link) - ^ a b Young, John (November 24, 2006). "Surface imaging of Betelgeuse with COAST and the WHT". University of Cambridge. Retrieved 2007-06-21.
Images of hotspots on the surface of Betelgeuse taken at visible and infra-red wavelengths using high resolution ground-based interferometers
Cite error: The named reference "YOUNG" was defined multiple times with different content (see the help page). - ^ Perrin, Guy; Malbet, Fabien (2003). "Observing with the VLTI". EAS Publications Series. 6. Bibcode:2003EAS.....6D...3P. doi:10.1051/eas/20030601.
{{cite journal}}
: Unknown parameter|origin=
ignored (help)CS1 maint: multiple names: authors list (link) - ^ Worden, S. (April 1978). "Speckle interferometry". New Scientist. 78: 238–40. Bibcode:1978NewSc..78..238W.
{{cite journal}}
: Unknown parameter|origin=
ignored (help)CS1 maint: date and year (link) - ^ Ron Cowen (2009-06-10). "Betelgeuse shrinks: The red supergiant has lost 15 percent of its size".
The shrinkage corresponds to the star contracting by a distance equal to that between Venus and the sun, researchers reported June 9 at an American Astronomical Society meeting and in the June 1 Astrophysical Journal Letters.
— Townes, C. H.; Wishnow, E. H.; Hale, D. D. S.; Walp, B. (2009). "A Systematic Change with Time in the Size of Betelgeuse". Astrophysical Journal Letters. 697 (2): L127. Bibcode:2009ApJ...697L.127T. doi:10.1088/0004-637X/697/2/L127. S2CID 121009337. - ^ Maeder, André; Meynet, Georges (2003). "The role of rotation and mass loss in the evolution of massive stars" (PDF). Astronomical Society of the Pacific. 212: 267. Bibcode:2003IAUS..212..267M. Retrieved 2007-08-16.
{{cite journal}}
: Unknown parameter|origin=
ignored (help)CS1 maint: multiple names: authors list (link) - ^ a b c Bernat, A. P. (1977). "The circumstellar shells and mass loss rates of four M supergiants". Astrophysical Journal. 213: 756–66. Bibcode:1977ApJ...213..756B. doi:10.1086/155205. S2CID 121146305.
- ^
Adams, Walter S.; MacCormack, Elizabeth (1935). "Systematic Displacements of Lines in the Spectra of Certain Bright Stars". Astrophysical Journal. 81: 119. Bibcode:1935ApJ....81..119A. doi:10.1086/143620.
{{cite journal}}
: CS1 maint: multiple names: authors list (link) - ^
Deutsch, Armin J. (1956). "The Circumstellar Envelope of Alpha Herculis". Astrophysical Journal. 123: 210. Bibcode:1956ApJ...123..210D. doi:10.1086/146152.
In the concluding sentence of the abstract, Deutsch notes that: This process may be important in the evolution of all massive stars that have exhausted their hydrogen—a reference to α Ori and other red giants experiencing mass loss.
- ^ a b "Sharpest views of Betelgeuse reveal how supergiant stars lose mass: Unveiling the true face of a behemoth". 29 July 2009. Retrieved 18 April 2010.
- ^ http://www.universetoday.com/guide-to-space/stars/betelgeuse/
- ^ Wheeler, J. Craig (2007). Cosmic Catastrophes: Exploding Stars, Black Holes, and Mapping the Universe (2nd ed.). Cambridge, UK: Cambridge University Press. pp. 115–17. ISBN 978-0-521-85714-7.
- ^ Dupree, Andrea K. (1987). "Monitoring the Variable Atmosphere of Alpha Orionis". IUE Proposal ID #LSJAD: 2758. Bibcode:1987iue..prop.2758D.