User:Isntthatabitlazy?/sandbox
![]() | This article needs attention from an expert in MCB. Please add a reason or a talk parameter to this template to explain the issue with the article.(August 2012) |

Schematic diagram of the basic Trimeric Autotransporter Adhesin structure

The C-terminal membrane anchor domain can clearly be seen on the right in blue. The stalk domain can be seen in red.
In molecular biology, trimeric autotransporter adhesins shortened to TAAs, are proteins found on the outer membrane of Gram-negative bacteria. Bacteria use TAAs in order to infect their host cells via a process called cell adhesion.[1] TAAs also go by another name, oligomeric coiled-coil adhesins, which is shortened to OCAs. In essence, they are virulence factors, factors that make the bacteria harmful and infective to the host organism.[2]
TAAs are just one of many methods bacteria use to infect their hosts, infection resulting in diseases such as pneumonia, sepsis, and meningitis. Most bacteria infect their host through a method named the secretion pathway. TAAs are part of the secretion pathway, to be more specific the type Vc secretion system.[3]
Trimeric autotransporter adhesins have a unique structure. The structure they hold is crucial to their function. They all appear to have a head-stalk-anchor structure. Each TAA is made up of three identical proteins, hence the name trimeric. Once the membrane anchor has been inserted into the outer membrane, the passenger domain passes through it into the host extracellular environment autonomously, hence the description of autotransporter. The head domain, once assembled, then adheres to an element of the host extracellular matrix, for example, collagen, fibronectin, etc.[2]
Molecular structure
[edit]Trimeric Autotransporter Adhesins are structurally complex, diverse proteins of the outer membrane. The first TAAs observed in electron microscopy were desribed as having a "lollipop" shape, consisting of an N-terminal head, a more slender stalk, and a C-terminal membrane anchor. While this is true for simple TAAs, architecturally more complex TAAs may alternate once or multiply between head and stalk segments. Hence, they strongly vary in length: the sizes of observed TAAs ranges over almost two orders of magnitude, between little more than 100 amino acids to roughly 10,000 amino acids. In addition, the segments, both head and stalk, consist of one or more domains or motifs that are specific to this segment type, and create the TAA in a assembly kit like style. Except for the anchor, however, no domain is ubiquitously present in TAAs (the anchor is the only homologous domain of TAAs); thus the anchor domain is used to define family of trimeric autotransporters. [4]

The protein domain arrangement of the Trimeric Autotransporter Adhesin, BadA[1] This figure shows the head, stalk and anchor domains. It shows the YadA-like head in grey. The stalk contains repeats coloured in green and the membrane anchor in red. The sequence below shows colouring according to domain arrangement and protease cleavage sites red (trypsin) and blue (chymotrypsin). (Figure used from open access journal, in the public domain, Public Library of Science (PLoS) Pathogen
Extended Signal Peptide Region domain
[edit]ESPR | |||||||||
---|---|---|---|---|---|---|---|---|---|
Identifiers | |||||||||
Symbol | ESPR | ||||||||
Pfam | PF13018 | ||||||||
|
The Extended Signal Peptide Region (ESPR) is found in the N-terminus of the signal peptides of proteins belonging to the Type V secretion systems. The function of the ESPR is to aid inner membrane translocation[5] by acting as a temporary tether. This prevents the accumulation of misfolded proteins.[6] The ESPR can be divided into individual regions, they are as follows: N1 (charged), H1 (hydrophobic), N2, H2 and C (cleavage site) domains. N1 and H1 form the ESPR and have strong conservation.[7]
Function: There are several roles that the Extended Signal Peptide Region is thought to hold. First, biogenesis of proteins in the Type V Secretion System (T5SS). Second, it is thought to target the protein to the inner membrane to be translocated either by the signal recognition particle pathway (SRP) or by twin arginine translocated (TAT). Third, it has been observed and believed to regulate the rate of protein migration into the periplasm.[7]
N-terminal head domain
[edit]Structure: This particular domain is a trimer of single-stranded, left-handed beta-helices. These associate to form a nine-coiled left-handed beta-roll.[8] It contains sequence motifs, of which there is a strong similarity with other TAA heads. This indicates that there is a lot of similarity when comparing protein structure. The head domain is connected to the stalk by a short, highly conserved sequence, which is often called the neck, or occasionally named the connector.[2]
Function: The function of this protein domain is to bind to the extracellular matrix of the host, most notably fibronectin, collagen, and laminin.[9] The head domain is very important for attachment to the host cell and for autoagglutination, sticking to itself.[1]

Comparison of Head domains in different Trimeric Autotransporter Adhesins[10] (Figure used from open access journal, in the public domain, Public Library of Science (PLoS) Pathogen)
There are several types of head domain.[11] Each domain helps the head to bind to a different component of the extracellular matrix. These are as follows: YadA-like head domain, Trp-ring, GIN, FxG, HIN1, and HIN2. This entry focuses on the first three mentioned.
YadA-like head
[edit]YadA-like head[12] is composed of single-stranded, left-handed beta-helices, which associate further to create a nine-coiled left-handed parallel beta-roll (LPBR). It is the tightest beta-roll structure known, and the first to be discovered. The YadA head domain has eight repeat motifs, each fourteen residues in length.[8][13][14]
Trp ring
[edit]The Trp ring[15] is the second-most-common TAA head. Trp is an amino acid named tryptophan. The Trp ring obtains its name from the high levels of tryptophan found in the C-terminal part of the Head domain.[16] These work by stabilising the transition between the coiled-coil and the beta-meander where the head meets the neck or stalk. In many cases, the Trp ring is often followed by the GIN domain.
GIN
[edit]The GIN domain[17] is a head domain named after its sequence motif GIN (Glycine-Isoleucine-Aspargine) motif. It has an all-beta structure, whereby the two pairs of antiparallel beta sheets are connected by a diagonally running extended beta-sheet. The sheets then further fold to form a beta prism in which each wall is composed of a complete set of five beta-strands.[16] The GIN domain is often followed by a neck domain.
Neck domain
[edit]Structure: The neck domain is a homotrimer, where three of the same subunits associate. All three subunits are arranged in such a way that they resemble a "safety pin"-like structure.[8]
Function: The function of the neck domain is to be the adaptor between the larger diameter of the beta-helices and the smaller one of the coiled coil.[2] Furthermore, just like its safety pin structure, it also has a function of pinning all three monomers together and pins it to the head domain.[8] This increases the stability of the neck domain.
There are seven different type of neck domains.[11] They are as follows: ISneck1, ISneck2, HANS connector, DALL-1, DALL-2, DALL-3, and the neck domain. This entry focuses on the ISneck domain.
ISneck domain
[edit]The ISneck domain is a type of neck domain. There are two types of ISneck domain. This first is an ISneck which is interrupted by an insertion. The insertion can take form of either folded (ISneck 1[18]) or much shorter, unfolded (ISneck 2[19]) perturbation.[16]
Stalk domain
[edit]YadA_stalk | |||||||||
---|---|---|---|---|---|---|---|---|---|
Identifiers | |||||||||
Symbol | YadA_stalk | ||||||||
Pfam | PF05662 | ||||||||
InterPro | IPR008635 | ||||||||
SCOP2 | 1s7m / SCOPe / SUPFAM | ||||||||
|
Structure: These domains are fibrous and found in highly repetitive numbers. They contain coiled coils and their length tends to vary among different species. The coiled-coil segments of the stalk domains have two unusual properties:
- they alternate from right-handed to left-handed supercoiling
- often interrupted by small globular domains, which owes to their appearance of segmented ropes.[2]
Furthermore, the stalk is made up entirely of pentadecads. Hence, the stalk domains can be considered alpha helical coiled-coils that deviate from the standard model due to their unusual properties.[20] From a deeper structural perspective, coiled-coil arranges itself in such a way that the crossing angle between the helices is almost zero. The packing of these helices follows a "knobs-into-holes" arrangement whereby hydrophobic residues protrude forming knobs that pack into cavities formed by other residues on another helix. Then, once the knobs are packed into cavities, the three helices are wound in register around each other, so all of the residues in certain positions are at the same height.[16]
Function: Their role is to act as spacers by moving the head domains away from the bacterial cell surface and toward the extracellular matrix of the host. They also have a role in protecting the bacterial cell against host defences.[2] They do this by aiding complement resistance. The stalk protein domain is also alternatively named the internal passenger domain.[21]
There are two types of stalk domain:[11] the FGG domain and the right-handed stalk domain.
C-terminal membrane anchor domain
[edit]YadA bacterial adhesin anchor domain | |||||||||
---|---|---|---|---|---|---|---|---|---|
![]() The beta barrel structure found in the C-terminus of the bacterial adhesin anchor domain, YadA[22] | |||||||||
Identifiers | |||||||||
Symbol | YadA_anchor | ||||||||
Pfam | PF03895 | ||||||||
Pfam clan | CL0327 | ||||||||
InterPro | IPR005594 | ||||||||
|
Structure: The structure of this protein domain is a left-handed coiled-coil followed by four transmembrane beta strands. It is thought that, once trimerisation has occurred, these beta strands further fold into a 12-stranded beta-barrel. It also contains a recognition site for signal peptidases, which means the enzyme will recognise the signal peptide and cleave it at a particular point.[6]
Function: The function of the membrane anchor domain is to aid the movement of the polypeptide chain through the cell membrane, a process known as autotransport activity.[2] The way TAAs translocate across the outer membrane remains to be elucidated, but it is thought that it translocates inside the beta-barrel, leading to transportation of the passenger domain from the C terminus to the N terminus through the beta-barrel lumen.[3] In essence, the beta-barrel is a porin that sits within the bacterial outer membrane. The passenger domain or in other words coiled-coil stalk domain translocates through this pore. Additional functions of the membrane anchor is to oligomerise the stalk domain and to anchor the whole protein to the bacterial outer membrane.[23]
Model proteins
[edit]All Trimeric Autotransporter Adhesins arecrucial virulence factors that cause serious disease in humans. The most-studied and well-known Trimeric Autotransporter Adhesins are listed below:[1]
- YadA of Yersinia enterocolitica
- NadA of Neisseria meningitidis
- UspA1 and A2 of Moraxella catarrhalis
- Hia and Hsf of Haemophilus influenzae
- BadA of Bartonella henselae
Domain | Proteins | |||||
---|---|---|---|---|---|---|
YadA | NadA | UspA1 | HadA | Hia | BadA | |
N terminal Head | Single-stranded, left-handed beta helix[9] | Globular head[24] | Beta propeller head[25] | Not present | Beta prisms | Similar to YadA head, contains left handed beta helix |
Neck | Present | Not present | Present | Present | Present | Present |
Stalk | Right-handed coiled-coil | Coiled-coil alpha helices followed by a linker region | Extended coiled-coil | Three-alpha helix coiled-coil | Three-alpha helix coiled-coil | Extended coiled-coil |
C terminal Membrane anchor | Beta barrel structure | Beta barrel structure | Beta barrel structure | Beta barrel structure | Beta barrel structure | Beta barrel structure |
YadA head domain | |||||||||
---|---|---|---|---|---|---|---|---|---|
![]() Crystal structure of the collagen-binding domain of Yersinia adhesin YadA | |||||||||
Identifiers | |||||||||
Symbol | YadA_head | ||||||||
Pfam | PF05658 | ||||||||
InterPro | IPR008640 | ||||||||
SCOP2 | 1p9h / SCOPe / SUPFAM | ||||||||
|
YadA protein
[edit]YadA is a protein domain found in Gram-negative bacteria such as Yersinia enterocolitica, Yersinia pestis, and Yersinia pseudotuberculosis. YadA stands for Yersinia adhesin protein A. This protein domain is an example of Trimeric Autotransporter Adhesins, and it was the first TAA to be discovered.[26] Like other TAAs, YadA also undergoes homotrimerisation to form a stable collagen-binding protein.[8] Homotrimerisation is a process whereby three of the same subunits, associate to make a complex of three identical YadA proteins. Furthermore, just like other TAAs, it has a head-stalk-anchor protein architecture.[9] The majority of TAAs share strong similarity in the C-terminal membrane anchor region, the only member to differ across TAAs is the head, neck, and stalk regions. The head region of YadA is composed of beta-helices further folded to create a nine-coiled left-handed parallel beta-roll (LPBR).[8]
NadA protein
[edit]Another example of a TAA is the NadA protein. The NadA protein is found in a species of Gram-negative bacteria called Neisseria meningitidis, which causes sepsis and meningitis in humans.[27] Studies have shown that the globular N-terminal head domain of NadA is vital for adhesion. NadA also contains a coiled-coil region and also a C-terminal membrane anchor.[24]
UspA1 protein
[edit]UspA1 is another example of a Trimeric Autotransporter Adhesin found in the bacterium Moraxella catarrhalis, found as a common cause of middle ear infections in humans. The structure of UspA1 also has a head domain at N-terminal domain, however it is folded into the beta propeller. Like the other TAAs, it has a coiled-coil stalk region but, in this case it is extended, and it has the TAA typical C-terminal beta barrel membrane anchor domain.[25]
Hia protein
[edit]The Hia protein is a TAA found on the outer membrane of the bacterium Haemophilus influenzae. It adheres to the respiratory epithelium in humans.[28] This protein can cause pneumonia and some strains cause meningitis and sepsis.[29] Hia has a slightly unusual N-terminal head made of beta-prisms. The beta-prism is an unusual type of protein architecture first described by Chothia and Murzin.[30] As the name suggests, it holds three beta sheets arranged in a triangular prism and contains internal symmetry.[31] Additionally, the head domain contains 5 Trp-Ring domains. Furthermore, this protein also contains three neck domains, of which two are IsNeck domains in addition to other domains such as KG, GANG, and TTT domains.[29] It also contains a coiled-coil stalk and the typically conserved TAA C terminal membrane anchor.[32]
BadA protein
[edit]The BadA protein is another example of a TAA found in Bartonella henselae bacteria. Bartonella henselae is the causative agent of cat scratch disease, a normally harmless disease, but, in people with a weakened immune system, such as those undergoing chemotherapy or fighting AIDS, it is more serious as it can lead to bacillary angiomatosis.[1] This a condition where benign tumours of the blood vessels undergo uncontrolled proliferation, causing knots to form in the smaller blood vessels, such as capillaries, restricting the flow of blood. This may be due to BadA's inducing the transcription of proangiogenic factors, as it activates of NF-κB as well as hypoxia-inducible factor 1.[33] The head domain of BadA is more complex than other TAAs. It is thought to be a chimera or, in other words, a combination of YadA and Hia head domains.[1] This combination gives insight into how the pathogenicity of Gram-negative bacteria has evolved over time. BadA also contains a neck domain, an extended coil-coil stalk, and beta-barrel C terminal membrane anchor.
Clinical effects
[edit]Protein Domain | Bacterial species | Diseases caused |
---|---|---|
YadA | Yersinia enterocolitica | yersiniosis |
NadA | Neisseria meningitidis | sepsis and meningitis |
UspA1 | Moraxella catarrhalis | middle ear infection |
Hia | Haemophilus influenzae | pneumonia and some strains cause meningitis and sepsis |
BadA | Bartonella henselae | cat scratch disease |
Infection Process
[edit]The process of infection is complicated. The invasive bacterium must overcome many barriers in order to infect its host, including environmental barriers, physical barriers and immune system barriers. The bacterium must enter the host's body and, in the case of Yersinia sp., invade the host intestinal mucosa. Then the Trimeric Autotransporter Adhesin must adhere to the layer of cells found on the internal surface, the epithelial cells, in the intestine by using its head to bind to proteins found in the extracellular matrix such as collagen, laminin, and fibronectin.[8] It is important that these outer-membrane adhesins make physical contact with the receptors found on the host cell. This means that the adhesin must be long enough to extend beyond the lipopolysaccharide layer in the outer membrane of the bacterium and interact with the glycan layer of the host cell.[29] Once it has done so, it may bind to the ECM of the host cell. TAAs are a type of microbial surface components recognizing adhesive matrix molecules (MSCRAMMs). In other words, they are a complex that aids adhesion to the ECM.[34]
Type V secretion system (T5SS)
[edit]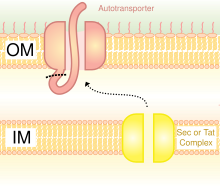
A schematic diagram illustrating the Trimeric Autotransporter Adhesins in Type V Secretion System.
Secretion is one method of transferring substances across the bacterial outer membrane. Gram-negative bacteria have very different cell wall structures in comparison to Gram-positive bactera. Gram-negative bacteria have three layers: The innermost layer is named the inner membrane; the middle layer, named the periplasmic space, is a space containing a thin layer of peptidoglycan; and the third layer is named the outer membrane, which contains lipopolysaccharides.[23]
In Gram-negative bacteria, the secretary pathway is very different from that of eukaryotes or Gram-positive bacteria, mainly due to the difference in cell wall structure.[35] Trimeric Autotransporter Adhesins use a particular secretion pathway, named type V secretion system (T5SS). Gram-negative bacteria must secrete adhesins, since they have an outer membrane that makes it hard for them to stick to and infect the host. The outer membrane is useful, as it allows the bacteria to colonize, and adds another layer of protection. However, the outer membrane is a barrier for the secretion of proteins, and it requires energy to transport proteins across the outer membrane. Hence, the T5SS pathway overcomes this problem.[35]
T5SS uses Sec-machinery system to work. The enzyme Sec translocase is found to be present on the inner membrane. Such Sec-dependent systems do not need to use energy, unlike Sec-independent machinery, which uses other forms of energy such as adenosine triphosphate (ATP) or a proton gradient. Since it can transport things across the outer membrane without the need to generate a new form of energy, it earned the name autotransporter, since it transports proteins autonomously,[36] in other words, by itself.
The Sec-dependent system is divided into three pathways. TAAs are one of those pathways and also go by the name type Vc secretion pathway. The mechanism is split into two. First, the protein must move across the inner membrane or, in other words, translocate, in a Sec-dependent manner via the periplasm.[37] The signal peptide on the N-terminus acts as a temporary tether to hold it in place. Next, it must move to the outer membrane. The trimerisation aids translocation, and no translocation would occur without its beta-barrel membrane anchor.[3] The type V secretion system is described as non-fimbrious, meaning that the bacterial cells do not use long physical appendages named pili to attach to one another.[6]
Evading the host's immune system
[edit]The TAAs can help prevent the bacteria from being destroyed by the host's immune system. In particular in the case of certain Yersinia spp., the TAA YadA has a role in autoagglutination, serum resistance, complement inactivation, and phagocytosis resistance. All of these methods prevent the bacteria from being eliminated by the host and ensure its survival.[8]
See also
[edit]External links
[edit]References
[edit]- ^ a b c d e f Szczesny P, Linke D, Ursinus A, Bär K, Schwarz H, Riess TM; et al. (2008). Ghosh, Partho (ed.). "Structure of the head of the Bartonella adhesin BadA". PLoS Pathog. 4 (8): e1000119. doi:10.1371/journal.ppat.1000119. PMC 2483945. PMID 18688279.
{{cite journal}}
: Explicit use of et al. in:|author=
(help)CS1 maint: multiple names: authors list (link) CS1 maint: unflagged free DOI (link) - ^ a b c d e f g Linke D, Riess T, Autenrieth IB, Lupas A, Kempf VA (2006). "Trimeric autotransporter adhesins: variable structure, common function". Trends Microbiol. 14 (6): 264–70. doi:10.1016/j.tim.2006.04.005. PMID 16678419.
{{cite journal}}
: CS1 maint: multiple names: authors list (link) - ^ a b c Mikula KM, Leo JC, Łyskowski A, Kedracka-Krok S, Pirog A, Goldman A (2012). "The translocation domain in trimeric autotransporter adhesins is necessary and sufficient for trimerization and autotransportation". J Bacteriol. 194 (4): 827–38. doi:10.1128/JB.05322-11. PMC 3272944. PMID 22155776.
{{cite journal}}
: CS1 maint: multiple names: authors list (link) - ^ Szczesny P, Lupas A (2008). "Domain annotation of trimeric autotransporter adhesins--daTAA". Bioinformatics. 24 (10): 1251–6. doi:10.1093/bioinformatics/btn118. PMC 2373917. PMID 18397894.
- ^ Desvaux M, Scott-Tucker A, Turner SM, Cooper LM, Huber D, Nataro JP; et al. (2007). "A conserved extended signal peptide region directs posttranslational protein translocation via a novel mechanism". Microbiology. 153 (Pt 1): 59–70. doi:10.1099/mic.0.29091-0. PMID 17185535.
{{cite journal}}
: Explicit use of et al. in:|author=
(help)CS1 maint: multiple names: authors list (link) CS1 maint: unflagged free DOI (link) - ^ a b c Leyton DL, Rossiter AE, Henderson IR (2012). "From self sufficiency to dependence: mechanisms and factors important for autotransporter biogenesis". Nat Rev Microbiol. 10 (3): 213–25. doi:10.1038/nrmicro2733. PMID 22337167.
{{cite journal}}
: CS1 maint: multiple names: authors list (link) - ^ a b Desvaux M, Cooper LM, Filenko NA, Scott-Tucker A, Turner SM, Cole JA; et al. (2006). "The unusual extended signal peptide region of the type V secretion system is phylogenetically restricted". FEMS Microbiol Lett. 264 (1): 22–30. doi:10.1111/j.1574-6968.2006.00425.x. PMID 17020545.
{{cite journal}}
: Explicit use of et al. in:|author=
(help)CS1 maint: multiple names: authors list (link) - ^ a b c d e f g h Nummelin H, Merckel MC, Leo JC, Lankinen H, Skurnik M, Goldman A (2004). "The Yersinia adhesin YadA collagen-binding domain structure is a novel left-handed parallel beta-roll". EMBO J. 23 (4): 701–11. doi:10.1038/sj.emboj.7600100. PMC 381008. PMID 14765110.
{{cite journal}}
: CS1 maint: multiple names: authors list (link) - ^ a b c Koretke KK, Szczesny P, Gruber M, Lupas AN (2006). "Model structure of the prototypical non-fimbrial adhesin YadA of Yersinia enterocolitica". J Struct Biol. 155 (2): 154–61. doi:10.1016/j.jsb.2006.03.012. PMID 16675268.
{{cite journal}}
: CS1 maint: multiple names: authors list (link) - ^ Edwards TE, Phan I, Abendroth J, Dieterich SH, Masoudi A, Guo W; et al. (2010). Kursula, Petri (ed.). "Structure of a Burkholderia pseudomallei trimeric autotransporter adhesin head". PLoS ONE. 5 (9): e12803. doi:10.1371/journal.pone.0012803. PMC 2942831. PMID 20862217.
{{cite journal}}
: Explicit use of et al. in:|author=
(help)CS1 maint: multiple names: authors list (link) CS1 maint: unflagged free DOI (link) - ^ a b c http://toolkit.tuebingen.mpg.de/dataa/browse[full citation needed]
- ^ http://toolkit.tuebingen.mpg.de/dataa/browse?page=show&domain=20&db=domains[full citation needed]
- ^ Valle J, Mabbett AN, Ulett GC, Toledo-Arana A, Wecker K, Totsika M; et al. (2008). "UpaG, a new member of the trimeric autotransporter family of adhesins in uropathogenic Escherichia coli". J Bacteriol. 190 (12): 4147–61. doi:10.1128/JB.00122-08. PMC 2446758. PMID 18424525.
{{cite journal}}
: Explicit use of et al. in:|author=
(help)CS1 maint: multiple names: authors list (link) - ^ Caserta R, Takita MA, Targon ML, Rosselli-Murai LK, de Souza AP, Peroni L; et al. (2010). "Expression of Xylella fastidiosa fimbrial and afimbrial proteins during biofilm formation". Appl Environ Microbiol. 76 (13): 4250–9. doi:10.1128/AEM.02114-09. PMC 2897468. PMID 20472735.
{{cite journal}}
: Explicit use of et al. in:|author=
(help)CS1 maint: multiple names: authors list (link) - ^ http://toolkit.tuebingen.mpg.de/dataa/browse?page=show&domain=2&db=domains[full citation needed]
- ^ a b c d Łyskowski A, Leo JC, Goldman A (2011). "Structure and biology of trimeric autotransporter adhesins". Adv Exp Med Biol. Advances in Experimental Medicine and Biology. 715: 143–58. doi:10.1007/978-94-007-0940-9_9. ISBN 978-94-007-0939-3. PMID 21557062.
{{cite journal}}
: CS1 maint: multiple names: authors list (link) - ^ http://toolkit.tuebingen.mpg.de/dataa/browse?page=show&domain=11&db=domains[full citation needed]
- ^ http://toolkit.tuebingen.mpg.de/dataa/browse?page=show&domain=16&db=domains[full citation needed]
- ^ http://toolkit.tuebingen.mpg.de/dataa/browse?page=show&domain=22&db=domains[full citation needed]
- ^ Lupas AN, Gruber M (2005). "The structure of alpha-helical coiled coils". Adv Protein Chem. Advances in Protein Chemistry. 70: 37–78. doi:10.1016/S0065-3233(05)70003-6. ISBN 9780120342709. PMID 15837513.
- ^ Cotter, S. E.; Surana, N. K.; St. Geme, J. W. (2005). "Trimeric autotransporters: A distinct subfamily of autotransporter proteins". Trends in Microbiology. 13 (5): 199–205. doi:10.1016/j.tim.2005.03.004. PMID 15866036.
- ^ http://www.ebi.ac.uk/thornton-srv/databases/cgi-bin/pdbsum/GetPage.pl[full citation needed]
- ^ a b Białas N, Kasperkiewicz K, Radziejewska-Lebrecht J, Skurnik M (2012). "Bacterial cell surface structures in Yersinia enterocolitica". Arch Immunol Ther Exp (Warsz). 60 (3): 199–209. doi:10.1007/s00005-012-0168-z. PMID 22484801.
{{cite journal}}
: CS1 maint: multiple names: authors list (link) - ^ a b Tavano R, Capecchi B, Montanari P, Franzoso S, Marin O, Sztukowska M; et al. (2011). "Mapping of the Neisseria meningitidis NadA cell-binding site: relevance of predicted {alpha}-helices in the NH2-terminal and dimeric coiled-coil regions". J Bacteriol. 193 (1): 107–15. doi:10.1128/JB.00430-10. PMC 3019930. PMID 20971901.
{{cite journal}}
: Explicit use of et al. in:|author=
(help)CS1 maint: multiple names: authors list (link) - ^ a b Agnew C, Borodina E, Zaccai NR, Conners R, Burton NM, Vicary JA; et al. (2011). "Correlation of in situ mechanosensitive responses of the Moraxella catarrhalis adhesin UspA1 with fibronectin and receptor CEACAM1 binding". Proc Natl Acad Sci U S A. 108 (37): 15174–8. doi:10.1073/pnas.1106341108. PMC 3174611. PMID 21876142.
{{cite journal}}
: Explicit use of et al. in:|author=
(help)CS1 maint: multiple names: authors list (link) - ^ Casutt-Meyer S, Renzi F, Schmaler M, Jann NJ, Amstutz M, Cornelis GR (2010). Bereswill, Stefan (ed.). "Oligomeric coiled-coil adhesin YadA is a double-edged sword". PLoS ONE. 5 (12): e15159. doi:10.1371/journal.pone.0015159. PMC 2999546. PMID 21170337.
{{cite journal}}
: CS1 maint: multiple names: authors list (link) CS1 maint: unflagged free DOI (link) - ^ Comanducci M, Bambini S, Brunelli B, Adu-Bobie J, Aricò B, Capecchi B; et al. (2002). "NadA, a novel vaccine candidate of Neisseria meningitidis". J Exp Med. 195 (11): 1445–54. doi:10.1084/jem.20020407. PMC 2193550. PMID 12045242.
{{cite journal}}
: Explicit use of et al. in:|author=
(help)CS1 maint: multiple names: authors list (link) - ^ Meng G, Surana NK, St Geme JW, Waksman G (2006). "Structure of the outer membrane translocator domain of the Haemophilus influenzae Hia trimeric autotransporter". EMBO J. 25 (11): 2297–304. doi:10.1038/sj.emboj.7601132. PMC 1478200. PMID 16688217.
{{cite journal}}
: CS1 maint: multiple names: authors list (link) - ^ a b c Meng G, St Geme JW, Waksman G (2008). "Repetitive architecture of the Haemophilus influenzae Hia trimeric autotransporter". J Mol Biol. 384 (4): 824–36. doi:10.1016/j.jmb.2008.09.085. PMC 2597055. PMID 18948113.
{{cite journal}}
: CS1 maint: multiple names: authors list (link) - ^ Andreeva A, Murzin AG (2010). "Structural classification of proteins and structural genomics: new insights into protein folding and evolution". Acta Crystallogr Sect F Struct Biol Cryst Commun. 66 (Pt 10): 1190–7. doi:10.1107/S1744309110007177. PMC 2954204. PMID 20944210.
- ^ Shimizu T, Morikawa K (1996). "The beta-prism: a new folding motif". Trends Biochem Sci. 21 (1): 3–6. doi:10.1016/s0968-0004(06)80018-6. PMID 8848836.
- ^ Surana NK, Cutter D, Barenkamp SJ, St Geme JW (2004). "The Haemophilus influenzae Hia autotransporter contains an unusually short trimeric translocator domain". J Biol Chem. 279 (15): 14679–85. doi:10.1074/jbc.M311496200. PMID 14726537.
{{cite journal}}
: CS1 maint: multiple names: authors list (link) CS1 maint: unflagged free DOI (link) - ^ Harms A, Dehio C (2012). "Intruders below the radar: molecular pathogenesis of Bartonella spp". Clin Microbiol Rev. 25 (1): 42–78. doi:10.1128/CMR.05009-11. PMC 3255967. PMID 22232371.
- ^ Harris LG, Richards RG (2006). "Staphylococci and implant surfaces: a review". Injury. 37 Suppl 2 (2): S3–14. doi:10.1016/j.injury.2006.04.003. PMID 16651069.
- ^ a b Gerlach RG, Hensel M (2007). "Protein secretion systems and adhesins: the molecular armory of Gram-negative pathogens". Int J Med Microbiol. 297 (6): 401–15. doi:10.1016/j.ijmm.2007.03.017. PMID 17482513.
- ^ Leo JC, Grin I, Linke D (2012). "Type V secretion: mechanism(s) of autotransport through the bacterial outer membrane". Philos Trans R Soc Lond B Biol Sci. 367 (1592): 1088–101. doi:10.1098/rstb.2011.0208. PMC 3297439. PMID 22411980.
{{cite journal}}
: CS1 maint: multiple names: authors list (link) - ^ Kostakioti M, Newman CL, Thanassi DG, Stathopoulos C (2005). "Mechanisms of protein export across the bacterial outer membrane". J Bacteriol. 187 (13): 4306–14. doi:10.1128/JB.187.13.4306-4314.2005. PMC 1151778. PMID 15968039.
{{cite journal}}
: CS1 maint: multiple names: authors list (link)
Category:Protein families Category:Protein domains Category:Virulence factors Category:Gram-negative bacteria Category:Secretion Category:Membrane proteins