Junk DNA
Junk DNA (non-functional DNA) is a DNA sequence that has no known biological function.[1][2] Most organisms have some junk DNA in their genomes—mostly, pseudogenes and fragments of transposons and viruses—but it is possible that some organisms have substantial amounts of junk DNA.[3]
All protein-coding regions are generally considered to be functional elements in genomes. Additionally, non-protein coding regions such as genes for ribosomal RNA and transfer RNA, regulatory sequences, origins of replication, centromeres, telomeres, and scaffold attachment regions are considered as functional elements. (See Non-coding DNA for more information.)
It is difficult to determine whether other regions of the genome are functional or nonfunctional. There is considerable controversy over which criteria should be used to identify function. Many scientists have an evolutionary view of the genome and they prefer criteria based on whether DNA sequences are preserved by natural selection.[4][5][6] Other scientists dispute this view or have different interpretations of the data.[7][8][9]
History
[edit]The idea that only a fraction of the human genome could be functional dates back to the late 1940s. The estimated mutation rate in humans suggested that if a large fraction of those mutations were deleterious then the human species could not survive such a mutation load (genetic load). This led to predictions in the late 1940s by one of the founders of population genetics, J.B.S. Haldane, and by Nobel laureate Hermann Muller, that only a small percentage of the human genome contains functional DNA elements (genes) that can be destroyed by mutation.[10][11] (see Genetic load for more information)
In 1966 Muller reviewed these predictions and concluded that the human genome could only contain about 30,000 genes based on the number of deleterious mutations that the species could tolerate.[12] Similar predictions were made by other leading experts in molecular evolution who concluded that the human genome could not contain more than 40,000 genes and that less than 10% of the genome was functional.[13][14][4][15]
The size of genomes in various species was known to vary considerably and there did not seem to be a correlation between genome size and the complexity of the species. Even closely related species could have very different genome sizes. This observation led to what came to be known as the C-value paradox.[16] The paradox was resolved with the discovery of repetitive DNA and the observation that most of the differences in genome size could be attributed to repetitive DNA.[16][17] Some scientists thought that most of the repetitive DNA was involved in regulating gene expression but many scientists thought that the excess repetitive DNA was nonfunctional.[18][16][19][20][21]

At about the same time (late 1960s) the newly developed technique of C0t analysis was refined to include RNA:DNA hybridization leading to the discovery that considerably less than 10% of the human genome was complementary to mRNA and this DNA was in the unique (non-repetitive) fraction. This confirmed the predictions made from genetic load arguments and was consistent with the idea that much of the repetitive DNA is nonfunctional.[22][23][24]
The idea that large amounts of eukaryotic genomes could be nonfunctional conflicted with the prevailing view of evolution in 1968 since it seemed likely that nonfunctional DNA would be eliminated by natural selection. The development of the neutral theory and the nearly neutral theory provided a way out of this problem since it allowed for the preservation of slightly deleterious nonfunctional DNA in accordance with fundamental principles of population genetics.[14][13][25]
The term "junk DNA" began to be used in the late 1950s[26] but Susumu Ohno popularized the term in a 1972 paper titled "So much 'junk' DNA in our genome"[27] where he summarized the current evidence that had accumulated by then.[27] In a second paper that same year, he concluded that 90% of mammalian genomes consisted of nonfunctional DNA.[4] The case for junk DNA was summarized in a lengthy paper by David Comings in 1972 where he listed four reasons for proposing junk DNA:[28]
- some organisms have a lot more DNA than they seem to require (C-value paradox),
- current estimates of the number of genes (in 1972) are much less than the number that can be accommodated,
- the mutation load would be too large if all the DNA were functional, and
- some junk DNA clearly exists.
The discovery of introns in the 1970s seemed to confirm the views of junk DNA proponents because it meant that genes were very large and even huge genomes could not accommodate large numbers of genes. The proponents of junk DNA tended to dismiss intron sequences as mostly nonfunctional DNA (junk) but junk DNA opponents advanced a number of hypotheses attributing functions of various sort to intron sequences.[29][30][31][32][33]
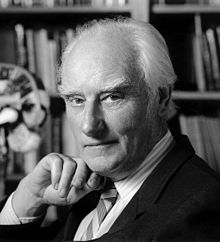
By 1980 it was apparent that most of the repetitive DNA in the human genome was related to transposons. This prompted a series of papers and letters describing transposons as selfish DNA that acted as a parasite in genomes and produced no fitness advantage for the organism.[34][35][36][37][38]
Opponents of junk DNA interpreted these results as evidence that most of the genome is functional and they developed several hypotheses advocating that transposon sequences could benefit the organism or the species.[39] The most important opponent of junk DNA at this time was Thomas Cavalier-Smith who argued that the extra DNA was required to increase the volume of the nucleus in order to promote more efficient transport across the nuclear membrane.[40]
The positions of the two sides of the controversy hardened with one side believing that evolution was consistent with large amounts of junk DNA and the other side believing that natural selection should eliminate junk DNA. These differing views of evolution were highlighted in a letter from Thomas Jukes, a proponent of junk DNA, to Francis Crick on December 20, 1979:[41]
"Dear Francis, I am sure that you realize how frightfully angry a lot of people will be if you say that much of the DNA is junk. The geneticists will be angry because they think that DNA is sacred. The Darwinian evolutionists will be outraged because they believe every change in DNA that is accepted in evolution is necessarily an adaptive change. To suggest anything else is an insult to the sacred memory of Darwin."
The other point of view was expressed by Roy John Britten and Kohne in their seminal paper on repetitive DNA.[17]
"A concept that is repugnant to us is that about half of the DNA of higher organisms is trivial or permanently inert (on an evolutionary timescale)."
Junk DNA and non-coding DNA
[edit]There is considerable confusion in the popular press and in the scientific literature about the distinction between non-coding DNA and junk DNA.
According to an article published in 2021 in American Scientist:
Close to 99 percent of our genome has been historically classified as noncoding, useless "junk" DNA. Consequently, these sequences were rarely studied.[42]
A book published in 2020 states:
When it was first discovered, the nongenic DNA was sometimes called—somewhat derisively by people who did not know better—"junk DNA" because it had no obvious utility, and they foolishly assumed that if it was not carrying coding information it must be useless trash.[43]
The common theme is that the original proponents of junk DNA thought that all non-coding DNA was junk.[2][6] This claim has been attributed to a paper by David Comings in 1972[28] where he is reported to have said that junk DNA refers to all non-coding DNA.[19] But Comings never said that. In that paper he discusses non-coding genes for ribosomal RNA and tRNAs and non-coding regulatory DNA and he proposes several possible functions for the bulk of non-coding DNA.[28] In another publication from the same year Comings again discusses the term junk DNA with the clear understanding that it does not include non-coding regulatory sequences.[44]
The idea that all non-coding DNA was thought to be junk has been criticized by numerous authors for distorting the history of junk DNA;[1][45][46][47][2] for example:
It is simply not true that noncoding DNA has long been dismissed as worthless junk and that functional hypotheses have only recently been proposed - despite the frequency with which this cliché is repeated in media reports and in the introduction of far too many scientific studies.[48]
Some of the criticisms have been strong:
Revisionist claims that equate noncoding DNA with junk merely reveal that people who are allowed to exhibit their logorrhea in Nature and other glam journals are as ignorant as the worst young-earth creationists.[49]
Functional vs non-functional
[edit]The main challenge of identifying junk DNA is to distinguish between "functional" and "non-functional " sequences. This is non-trivial, but there is some good evidence for both categories.
Functional
[edit]Protein-coding sequences are the most obvious functional sequences in genomes. However, they make up only 1-2% of most vertebrate genomes. However, there are also functional but non-coding DNA sequences[2] such as regulatory sequences, origins of replication, and centromeres.[50] These sequences are usually conserved in evolution and make up another 3-8% of the human genome.[51]
The Encyclopedia of DNA Elements (ENCODE) project reported that detectable biochemical activity was observed in regions covering at least 80% of the human genome, with biochemical activity defined primarily as being transcribed.[52] While these findings were announced as the demise of junk DNA[53][54] it is important to point out that transcription does not mean a sequence is "functional", analogous to some meaningless text that can be transcribed or copied without having any meaning.[55][1][56][57][58][2][46][59][5][45][9]
Non-functional
[edit]Non-functional DNA is rare in bacterial genomes which typically have an extremely high gene density, with only a few percent being not protein-coding.[60]
However, in most animal or plant genomes, a large fraction of DNA is non-functional, given that there is no obvious selective pressure on these sequences. More importantly, there is strong evidence that these sequences are not functional in other ways (using the human genome as example):
(1) Repetitive elements, especially mobile elements make up a large fraction of the human genome, such as LTR retrotransposons (8.3% of total genome), SINEs (13.1% of total genome) including Alu elements, LINEs (20.4% of total genome), SVAs (SINE-VNTR-Alu) and Class II DNA transposons (2.9% of total genome).[61] Many of these sequences are the descendents of ancient virus infections and are thus "non-functional" in terms of human genome function.
(2) Many sequences can be deleted as shown by comparing genomes. For instance, an analysis of 14,623 individuals identified 42,765 structural variants in the human genome of which 23.4% affected multiple genes (by deleting them or part of them). This study also found 47 deletions of >1 MB, showing that large chunks of the human genome can get deleted without obvious consequences.[62]
(3) Only a small fraction of the human genome is conserved, indicating that there is no strong (functional) selection pressure on these sequences, so they can rather freely mutate.[51][63] About 11% or less of the human genome is conserved[64][65] and about 7% is under purifying selection.[66]
Opponents of junk DNA argue that biochemical activity detects functional regions of the genome that are not identified by sequence conservation or purifying selection.[67][8][68] According to some scientists, until a region in question has been shown to have additional features, beyond what is expected of the null hypothesis, it should provisionally be labelled as non-functional.[69]
See also
[edit]References
[edit]- ^ a b c Eddy SR (November 2012). "The C-value paradox, junk DNA and ENCODE". Current Biology. 22 (21): R898–R899. Bibcode:2012CBio...22.R898E. doi:10.1016/j.cub.2012.10.002. PMID 23137679. S2CID 28289437.
- ^ a b c d e Palazzo AF, Gregory TR (May 2014). "The case for junk DNA". PLOS Genetics. 10 (5): e1004351. doi:10.1371/journal.pgen.1004351. PMC 4014423. PMID 24809441.
- ^ Gil R, Latorre A (October 2012). "Factors behind junk DNA in bacteria". Genes. 3 (4): 634–650. doi:10.3390/genes3040634. PMC 3899985. PMID 24705080.
- ^ a b c Ohno S (1972). "An argument for the genetic simplicity of man and other mammals". Journal of Human Evolution. 1 (6): 651–662. Bibcode:1972JHumE...1..651O. doi:10.1016/0047-2484(72)90011-5.
- ^ a b Morange M (2014). "Genome as a multipurpose structure built by evolution" (PDF). Perspectives in Biology and Medicine. 57 (1): 162–171. doi:10.1353/pbm.2014.0008. PMID 25345709. S2CID 27613442.
- ^ a b Palazzo AF, Kejiou NS (2022). "Non-Darwinian Molecular Biology". Frontiers in Genetics. 13: 831068. doi:10.3389/fgene.2022.831068. PMC 8888898. PMID 35251134.
- ^ Germain PL, Ratti E, and Boem F (2014). "Junk or functional DNA? ENCODE and the function controversy". Biology & Philosophy. 29 (6): 807–821. doi:10.1007/s10539-014-9441-3. S2CID 254277794.
- ^ a b Mattick JS (2023). "RNA out of the mist". Trends in Genetics. 39 (3): 187–207. doi:10.1016/j.tig.2022.11.001. PMID 36528415. S2CID 254768457.
- ^ a b Kellis M, Wold B, Snyder MP, Bernstein BE, Kundaje A, Marinov GK, et al. (April 2014). "Defining functional DNA elements in the human genome". Proceedings of the National Academy of Sciences of the United States of America. 111 (17): 6131–6138. Bibcode:2014PNAS..111.6131K. doi:10.1073/pnas.1318948111. PMC 4035993. PMID 24753594.
- ^ Muller HJ (June 1950). "Our load of mutations". American Journal of Human Genetics. 2 (2): 111–176. PMC 1716299. PMID 14771033.
- ^ Haldane J (1949). "The rate of mutation of human genes". Hereditas. 35: 267–273. doi:10.1111/j.1601-5223.1949.tb03339.x.
- ^ Lin Y, Zhai T, Zhang X (April 2014). "Nanoscale heat transfer in direct nanopatterning into gold films by a nanosecond laser pulse". Optics Express. 22 (7): 8396–8404. doi:10.1086/282445. JSTOR 2459205. PMID 24718213. S2CID 84202145.
- ^ a b Kimura M (February 1968). "Evolutionary rate at the molecular level". Nature. 217 (5129): 624–626. Bibcode:1968Natur.217..624K. doi:10.1038/217624a0. PMID 5637732. S2CID 4161261.
- ^ a b King JL, Jukes TH (May 1969). "Non-Darwinian evolution". Science. 164 (3881): 788–798. Bibcode:1969Sci...164..788L. doi:10.1126/science.164.3881.788. PMID 5767777.
- ^ Ohta T, Kimura M (September 1971). "Functional organization of genetic material as a product of molecular evolution". Nature. 233 (5315): 118–119. Bibcode:1971Natur.233..118O. doi:10.1038/233118a0. PMID 16063236. S2CID 13344748.
- ^ a b c Thomas CA (1971). "The genetic organization of chromosomes". Annual Review of Genetics. 5: 237–256. doi:10.1146/annurev.ge.05.120171.001321. PMID 16097657.
- ^ a b Britten RJ, Kohne DE (August 1968). "Repeated sequences in DNA. Hundreds of thousands of copies of DNA sequences have been incorporated into the genomes of higher organisms". Science. 161 (3841): 529–540. Bibcode:1968Sci...161..529B. doi:10.1126/science.161.3841.529. PMID 4874239.
- ^ Britten RJ, Davidson EH (July 1969). "Gene regulation for higher cells: a theory". Science. 165 (3891): 349–357. Bibcode:1969Sci...165..349B. doi:10.1126/science.165.3891.349. PMID 5789433.
- ^ a b Gregory TR (2005). "Genome Size Evolution in Animals". The Evolution of the Genome. Elsevier. pp. 3–87.
- ^ Lewin B (1974). "Chapter 4: Sequences of Eukaryotic DNA". Gene Expression-2: Eukaryotic Chromosomes. John Wiley & Sons.
- ^ Lewin B (1974). "Sequence Organization of Eukaryotic DNA: Defining the Unit of Gene Expression". Cell. 1 (3): 107–111. doi:10.1016/0092-8674(74)90125-1.
- ^ Lewin B (1974). "Chapter 5: Transcription and Processing of RNA". Gene Expression-2: Eukaryotic Chromosomes. John Wiley & Sons.
- ^ O'Brien SJ (March 1973). "On estimating functional gene number in eukaryotes". Nature. 242 (115): 52–54. doi:10.1038/newbio242052a0. PMID 4512011.
- ^ Bishop JO (June 1974). "The gene numbers game". Cell. 2 (2): 81–86. doi:10.1016/0092-8674(74)90095-6. PMID 4616752.
- ^ Kimura M, Ohta T (February 1971). "Protein polymorphism as a phase of molecular evolution". Nature. 229 (5285): 467–469. Bibcode:1971Natur.229..467K. doi:10.1038/229467a0. PMID 4925204. S2CID 4290427.
- ^ Sweet A (2022). Requiem for a Gene: The Problem of Junk DNA for the Molecular Paradigm (MA). University of Chicago.
- ^ a b Ohno S (1972). "So much "junk" DNA in our genome". Brookhaven Symposia in Biology. 23: 366–370. PMID 5065367.
- ^ a b c Comings DE (1972). "The structure and function of chromatin". Advances in human genetics. Springer. pp. 237–431.
- ^ Morange M (2020). "Chapter 17: Split Genes and Splicing". The Black Box of Biology: A History of the Molecular Revolution. Harvard University Press.
- ^ Gilbert W (February 1978). "Why genes in pieces?". Nature. 271 (5645): 501. Bibcode:1978Natur.271..501G. doi:10.1038/271501a0. PMID 622185. S2CID 4216649.
- ^ Gilbert W (May 1985). "Genes-in-pieces revisited". Science. 228 (4701): 823–824. Bibcode:1985Sci...228..823G. doi:10.1126/science.4001923. PMID 4001923.
- ^ Crick F (April 1979). "Split genes and RNA splicing". Science. 204 (4390): 264–271. Bibcode:1979Sci...204..264C. doi:10.1126/science.373120. PMID 373120.
- ^ Doolittle W (1978). "Genes in pieces: were they ever together?". Nature. 272 (5654): 581–582. Bibcode:1978Natur.272..581D. doi:10.1038/272581a0. S2CID 4162765.
- ^ Doolittle WF, Sapienza C (April 1980). "Selfish genes, the phenotype paradigm and genome evolution". Nature. 284 (5757): 601–603. Bibcode:1980Natur.284..601D. doi:10.1038/284601a0. PMID 6245369. S2CID 4311366.
- ^ Orgel LE, Crick FH (April 1980). "Selfish DNA: the ultimate parasite". Nature. 284 (5757): 604–607. Bibcode:1980Natur.284..604O. doi:10.1038/284604a0. PMID 7366731. S2CID 4233826.
- ^ Dover G (June 1980). "Ignorant DNA?". Nature. 285 (5767): 618–620. Bibcode:1980Natur.285..618D. doi:10.1038/285618a0. PMID 7393318. S2CID 4261755.
- ^ Dover G, Doolittle WF (December 1980). "Modes of genome evolution". Nature. 288 (5792): 646–647. Bibcode:1980Natur.288..646D. doi:10.1038/288646a0. PMID 6256636. S2CID 8938434.
- ^ Jain HK (December 1980). "Incidental DNA". Nature. 288 (5792): 647–648. Bibcode:1980Natur.288..647J. doi:10.1038/288647a0. PMID 7453799. S2CID 31899622.
- ^ Cavalier-Smith T (June 1980). "How selfish is DNA?". Nature. 285 (5767): 617–618. Bibcode:1980Natur.285..617C. doi:10.1038/285617a0. PMID 7393317. S2CID 27111068.
- ^ Cavalier-Smith T (December 1978). "Nuclear volume control by nucleoskeletal DNA, selection for cell volume and cell growth rate, and the solution of the DNA C-value paradox". Journal of Cell Science. 34: 247–278. doi:10.1242/jcs.34.1.247. PMID 372199.
- ^ Thomas J (December 29, 1979). "letter to Francis Crick". National Institutes of Health (USA).
- ^ Mortola E, Long M (2021). "Turning Junk into Us: How Genes Are Born". American Scientist. 109: 174–182.
- ^ McHughen A (2020). DNA Demystified: Unraveling the Double Helix. New York, New York, USA: Oxford University Press.
- ^ Comings DE (1972). "Review of Evolution of Genetics Systems". American Journal of Human Genetics. 25: 340–342.
- ^ a b Niu DK, Jiang L (2013). "Can ENCODE tell us how much junk DNA we carry in our genome?". Biochemical and Biophysical Research Communications. 430 (4): 1340–1343. doi:10.1016/j.bbrc.2012.12.074. PMID 23268340.
- ^ a b Graur D, Zheng Y, Price N, Azevedo RB, Zufall RA, Elhaik E (2013). "On the immortality of television sets: "function" in the human genome according to the evolution-free gospel of ENCODE". Genome Biology and Evolution. 5 (3): 578–590. doi:10.1093/gbe/evt028. PMC 3622293. PMID 23431001.
- ^ Graur D, Zheng Y, Azevedo RB (2015). "An evolutionary classification of genomic function". Genome Biology and Evolution. 7 (3): 642–645. doi:10.1093/gbe/evv021. PMC 5322545. PMID 25635041.
- ^ Elliott TA, Linquist S, and Gregory TR (2014). "Conceptual and empirical challenges of ascribing functions to transposable elements" (PDF). The American Naturalist. 184 (1): 14–24. doi:10.1086/676588. PMID 24921597. S2CID 14549993.
- ^ Graur D (2017). "Rubbish DNA: The functionless fraction of the human genome". In Saitou N (ed.). Evolution of the Human Genome I. Springer. pp. 19–60.
- ^ Watson J (1965). Molecular Biology of the Gene. New York, New York, USA: W. A. Benjamin, Inc.
- ^ a b Siepel A, Bejerano G, Pedersen JS, Hinrichs AS, Hou M, Rosenbloom K, et al. (August 2005). "Evolutionarily conserved elements in vertebrate, insect, worm, and yeast genomes". Genome Research. 15 (8): 1034–1050. doi:10.1101/gr.3715005. PMC 1182216. PMID 16024819.
- ^ Dunham I, Kundaje A, Aldred SF, Collins PJ, Davis CA, Doyle F, et al. (The ENCODE Project Consortium) (September 2012). "An integrated encyclopedia of DNA elements in the human genome". Nature. 489 (7414): 57–74. Bibcode:2012Natur.489...57T. doi:10.1038/nature11247. PMC 3439153. PMID 22955616.
- ^ Pennisi E (September 2012). "Genomics. ENCODE project writes eulogy for junk DNA". Science. 337 (6099): 1159, 1161. doi:10.1126/science.337.6099.1159. PMID 22955811.
- ^ Casane D, Fumey J, Laurenti P (2015). "[ENCODE apophenia or a panglossian analysis of the human genome]". Médecine/Sciences. 31 (6–7): 680–686. doi:10.1051/medsci/20153106023. PMID 26152174.
- ^ McKie R (February 24, 2013). "Scientists attacked over claim that 'junk DNA' is vital to life". The Observer.
- ^ Eddy SR (April 2013). "The ENCODE project: missteps overshadowing a success". Current Biology. 23 (7): R259–R261. Bibcode:2013CBio...23.R259E. doi:10.1016/j.cub.2013.03.023. PMID 23578867.
- ^ Doolittle WF (April 2013). "Is junk DNA bunk? A critique of ENCODE". Proceedings of the National Academy of Sciences of the United States of America. 110 (14): 5294–5300. Bibcode:2013PNAS..110.5294D. doi:10.1073/pnas.1221376110. PMC 3619371. PMID 23479647.
- ^ Brunet TD, Doolittle WF (August 2014). "Getting "function" right". Proceedings of the National Academy of Sciences of the United States of America. 111 (33): E3365. Bibcode:2014PNAS..111E3365P. doi:10.1073/pnas.1409762111. PMC 4143013. PMID 25107292.
- ^ Doolittle WF, Brunet TD, Linquist S, Gregory TR (May 2014). "Distinguishing between "function" and "effect" in genome biology". Genome Biology and Evolution. 6 (5): 1234–1237. doi:10.1093/gbe/evu098. PMC 4041003. PMID 24814287.
- ^ Zhao Z, Cristian A, Rosen G (September 2020). "Keeping up with the genomes: efficient learning of our increasing knowledge of the tree of life". BMC Bioinformatics. 21 (1): 412. doi:10.1186/s12859-020-03744-7. PMC 7507296. PMID 32957925.
- ^ Treangen TJ, Salzberg SL (November 2011). "Repetitive DNA and next-generation sequencing: computational challenges and solutions". Nature Reviews. Genetics. 13 (1): 36–46. doi:10.1038/nrg3117. PMC 3324860. PMID 22124482.
- ^ Abel HJ, Larson DE, Regier AA, Chiang C, Das I, Kanchi KL, et al. (July 2020). "Mapping and characterization of structural variation in 17,795 human genomes". Nature. 583 (7814): 83–89. Bibcode:2020Natur.583...83A. doi:10.1038/s41586-020-2371-0. PMC 7547914. PMID 32460305.
- ^ Graur D (2016). Molecular and Genome Evolution. Sunderland MA (USA): Sinauer Associates, Inc. ISBN 9781605354699.
- ^ Rands CM, Meader S, Ponting CP, Lunter G (July 2014). "8.2% of the Human genome is constrained: variation in rates of turnover across functional element classes in the human lineage". PLOS Genetics. 10 (7): e1004525. doi:10.1371/journal.pgen.1004525. PMC 4109858. PMID 25057982.
- ^ Christmas MJ, Kaplow IM, Genereux DP, Dong MX, Hughes GM, Li X, et al. (April 2023). "Evolutionary constraint and innovation across hundreds of placental mammals". Science. 380 (6643): eabn3943. doi:10.1126/science.abn3943. hdl:10230/59591. PMC 10250106. PMID 37104599.
- ^ Halldorsson BV, Eggertsson HP, Moore KH, Hauswedell H, Eiriksson O, Ulfarsson MO, et al. (July 2022). "The sequences of 150,119 genomes in the UK Biobank". Nature. 607 (7920): 732–740. Bibcode:2022Natur.607..732H. doi:10.1038/s41586-022-04965-x. hdl:20.500.11815/3726. PMC 9329122. PMID 35859178.
- ^ Liu G, Mattick JS, Taft RJ (July 2013). "A meta-analysis of the genomic and transcriptomic composition of complex life". Cell Cycle. 12 (13): 2061–2072. doi:10.1186/1877-6566-7-2. PMC 4685169. PMID 23759593.
- ^ Mattick JS (September 2023). "A Kuhnian revolution in molecular biology: Most genes in complex organisms express regulatory RNAs". BioEssays. 45 (9): e2300080. doi:10.1002/bies.202300080. PMID 37318305.
- ^ Palazzo AF, Lee ES (2015). "Non-coding RNA: what is functional and what is junk?". Frontiers in Genetics. 6: 2. doi:10.3389/fgene.2015.00002. PMC 4306305. PMID 25674102.