Brønsted–Lowry acid–base theory
The Brønsted–Lowry theory (also called proton theory of acids and bases[1]) is an acid–base reaction theory which was first developed by Johannes Nicolaus Brønsted and Thomas Martin Lowry independently in 1923.[2][3] The basic concept of this theory is that when an acid and a base react with each other, the acid forms its conjugate base, and the base forms its conjugate acid by exchange of a proton (the hydrogen cation, or H+). This theory generalises the Arrhenius theory.
Definitions of acids and bases
[edit]In the Arrhenius theory, acids are defined as substances that dissociate in aqueous solutions to give H+ (hydrogen ions or protons), while bases are defined as substances that dissociate in aqueous solutions to give OH− (hydroxide ions).[4]
In 1923, physical chemists Johannes Nicolaus Brønsted in Denmark and Thomas Martin Lowry in England both independently proposed the theory named after them.[5][6][7] In the Brønsted–Lowry theory acids and bases are defined by the way they react with each other, generalising them. This is best illustrated by an equilibrium equation.
- acid + base ⇌ conjugate base + conjugate acid.
With an acid, HA, the equation can be written symbolically as:
The equilibrium sign, ⇌, is used because the reaction can occur in both forward and backward directions (is reversible). The acid, HA, is a proton donor which can lose a proton to become its conjugate base, A−. The base, B, is a proton acceptor which can become its conjugate acid, HB+. Most acid–base reactions are fast, so the substances in the reaction are usually in dynamic equilibrium with each other.[8]
Aqueous solutions
[edit]
Consider the following acid–base reaction:
Acetic acid, CH3COOH, is an acid because it donates a proton to water (H2O) and becomes its conjugate base, the acetate ion (CH3COO−). H2O is a base because it accepts a proton from CH3COOH and becomes its conjugate acid, the hydronium ion, (H3O+).[9]
The reverse of an acid–base reaction is also an acid–base reaction, between the conjugate acid of the base in the first reaction and the conjugate base of the acid. In the above example, ethanoate is the base of the reverse reaction and hydronium ion is the acid.
One feature of the Brønsted–Lowry theory in contrast to Arrhenius theory is that it does not require an acid to dissociate.
Amphoteric substances
[edit]
The essence of Brønsted–Lowry theory is that an acid is only such in relation to a base, and vice versa. Water is amphoteric as it can act as an acid or as a base. In the image shown at the right one molecule of H2O acts as a base and gains H+ to become H3O+ while the other acts as an acid and loses H+ to become OH−.
Another example is illustrated by substances like aluminium hydroxide, Al(OH)3.
Non-aqueous solutions
[edit]The hydrogen ion, or hydronium ion, is a Brønsted–Lowry acid when dissolved in H2O and the hydroxide ion is a base because of the autoionization of water reaction
An analogous reaction occurs in liquid ammonia
Thus, the ammonium ion, NH+4, in liquid ammonia corresponds to the hydronium ion in water and the amide ion, NH−2 in ammonia, to the hydroxide ion in water. Ammonium salts behave as acids, and metal amides behave as bases.[10]
Some non-aqueous solvents can behave as bases, i.e. accept protons, in relation to Brønsted–Lowry acids.
where S stands for a solvent molecule. The most important of such solvents are dimethylsulfoxide, DMSO, and acetonitrile, CH3CN, as these solvents have been widely used to measure the acid dissociation constants of carbon-containing molecules. Because DMSO accepts protons more strongly than H2O the acid becomes stronger in this solvent than in water.[11] Indeed, many molecules behave as acids in non-aqueous solutions but not in aqueous solutions. An extreme case occurs with carbon acids, where a proton is extracted from a C−H bond.[12]
Some non-aqueous solvents can behave as acids. An acidic solvent will make dissolved substances more basic. For example, the compound CH3COOH is known as acetic acid since it behaves as an acid in water. However, it behaves as a base in liquid hydrogen fluoride, a much more acidic solvent.[13]
Comparison with Lewis acid–base theory
[edit]In the same year that Brønsted and Lowry published their theory, G. N. Lewis created an alternative theory of acid–base reactions. The Lewis theory is based on electronic structure. A Lewis base is a compound that can give an electron pair to a Lewis acid, a compound that can accept an electron pair.[14][15] Lewis's proposal explains the Brønsted–Lowry classification using electronic structure.
In this representation both the base, B, and the conjugate base, A−, are shown carrying a lone pair of electrons and the proton, which is a Lewis acid, is transferred between them.
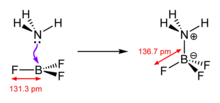
Lewis later wrote "To restrict the group of acids to those substances that contain hydrogen interferes as seriously with the systematic understanding of chemistry as would the restriction of the term oxidizing agent to substances containing oxygen."[15] In Lewis theory an acid, A, and a base, B, form an adduct, AB, where the electron pair forms a dative covalent bond between A and B. This is shown when the adduct H3N−BF3 forms from ammonia and boron trifluoride, a reaction that cannot occur in water because boron trifluoride hydrolizes in water.[16]
The reaction above illustrate that BF3 is an acid in both Lewis[17] and Brønsted–Lowry classifications and show that both theories agree with each other.[citation needed]
Boric acid is recognised as a Lewis acid because of the reaction
In this case the acid does not split up but the base, H2O, does. A solution of B(OH)3 is acidic because hydrogen ions are given off in this reaction.
There is strong evidence that dilute aqueous solutions of ammonia contain minute amounts of the ammonium ion
and that, when dissolved in water, ammonia functions as a Lewis base.[18]
Comparison with the Lux–Flood theory
[edit]The reactions between oxides in the solid or liquid states are excluded in the Brønsted–Lowry theory. For example, the reaction
is not covered in the Brønsted–Lowry definition of acids and bases. On the other hand, magnesium oxide acts as a base when it reacts with an aqueous solution of an acid.
Dissolved silicon dioxide, SiO2, has been predicted to be a weak acid in the Brønsted–Lowry sense.[19]
According to the Lux–Flood theory, oxides like MgO and SiO2 in the solid state may be called acids or bases. For example, the mineral olivine may be known as a compound of a basic oxide, MgO, and silicon dioxide, SiO2, as an acidic oxide. This is important in geochemistry.[citation needed]
References
[edit]- ^ "Brønsted–Lowry theory | chemistry". Encyclopedia Britannica. Retrieved 2021-03-07.
- ^ Brönsted, J. N. (1923). "Einige Bemerkungen über den Begriff der Säuren und Basen" [Some observations about the concept of acids and bases]. Recueil des Travaux Chimiques des Pays-Bas. 42 (8): 718–728. doi:10.1002/recl.19230420815.
- ^ Lowry, T. M. (1923). "The uniqueness of hydrogen". Journal of the Society of Chemical Industry. 42 (3): 43–47. doi:10.1002/jctb.5000420302.
- ^ Myers, Richard (2003). The Basics of Chemistry. Greenwood Publishing Group. pp. 157–161. ISBN 978-0-313-31664-7.
- ^ Masterton, William; Hurley, Cecile; Neth, Edward (2011). Chemistry: Principles and Reactions. Cengage Learning. p. 433. ISBN 978-1-133-38694-0.
- ^ Ebbing, Darrell; Gammon, Steven D. (2010). General Chemistry, Enhanced Edition. Cengage Learning. pp. 644–645. ISBN 978-0-538-49752-7.
- ^ Whitten, Kenneth; Davis, Raymond; Peck, Larry; Stanley, George (2013). Chemistry. Cengage Learning. p. 350. ISBN 978-1-133-61066-3.
- ^ Lew, Kristi (2009). Acids and Bases. Infobase Publishing. ISBN 9780791097830.
- ^ Patrick 2004, p. 76.
- ^ Holliday, A.K.; Massy, A.G. (1965). Inorganic Chemistry in Non-Aqueous Solvents. Pergamon Press.
- ^ Reich, Hans J. "Bordwell pKa Table (Acidity in DMSO)". Department of Chemistry, University of Wisconsin, U.S. Archived from the original on 9 October 2008. Retrieved 2008-11-02.
- ^ Smith, Michael; March, Jerry (2001). March's advanced organic chemistry: reactions, mechanisms, and structure (5th ed.). New York: Wiley. p. 328. ISBN 978-0-471-58589-3.
- ^ Waddington, T.C. (1965). Non-Aqueous Solvent Systems. New York: Academic Press. p. 69.
- ^ Miessler, G. L., Tarr, D. A., (1991) "Inorganic Chemistry" 2nd ed. Pearson Prentice-Hall pp. 170–172
- ^ a b Hall, Norris F. (March 1940). "Systems of Acids and Bases". Journal of Chemical Education. 17 (3): 124–128. Bibcode:1940JChEd..17..124H. doi:10.1021/ed017p124.
- ^ a b Wamser, Christian A. (1951-01-01). "Equilibria in the System Boron Trifluoride—Water at 25°". Journal of the American Chemical Society. 73 (1): 409–416. doi:10.1021/ja01145a134. ISSN 0002-7863.
- ^ Patrick 2004, p. 91.
- ^ Housecroft, C. E.; Sharpe, A. G. (2004). Inorganic Chemistry (2nd ed.). Prentice Hall. p. 187. ISBN 978-0-13-039913-7.
- ^ Pauling, Linus (1960). The Nature of the Chemical Bond (3rd ed.). Ithaca: Cornell University Press. p. 557.
Bibliography
[edit]- Stoker, H. Stephen (2012). General, Organic, and Biological Chemistry. Cengage Learning. ISBN 978-1-133-10394-3.
- Myers, Richard (2003). The Basics of Chemistry. Greenwood Publishing Group. ISBN 978-0-313-31664-7.
- Patrick, Graham (2004). Instant Notes in Organic Chemistry. Taylor & Francis. ISBN 978-1-135-32125-3.
- Srivastava, H. C. (2003). Nootan - ISC Chemistry (7 ed.). India: Nageen Prakashan. ISBN 978-93-80088-89-1.
- Ramakrishna, A. (2014). Goyal's IIT FOUNDATION COURSE CHEMISTRY: For Class-10. Goyal Brothers Prakashan. p. 85. GGKEY:DKWFNS6PECF.
- Masterton, William; Hurley, Cecile; Neth, Edward (2011). Chemistry: Principles and Reactions. Cengage Learning. ISBN 978-1-133-38694-0.
- Whitten, Kenneth; Davis, Raymond; Peck, Larry; Stanley, George (2013). Chemistry. Cengage Learning. ISBN 978-1-133-61066-3.
- Ebbing, Darrell; Gammon, Steven D. (2010). General Chemistry, Enhanced Edition. Cengage Learning. pp. 644–645. ISBN 978-0-538-49752-7.